2019 Seminar Archive
Spring, 2019
Active and Architectured Structures: From Nematic Elastomers Sheets to Rigidly-Foldable Origami.
Paul Plucinsky
Postdoctoral Researcher Department of Aerospace Engineering and Mechanics College of Science & Engineering Unversity of Minnesota, Twin Cities Minneapolis, MN
Thin and slender structures exhibit a broad range of mechanical responses as the competition between stretching and bending in these structures can result in buckling, localized deformations like folding, and tension wrinkling. Active and architectured materials also exhibit a broad range of mechanical responses as features that manifest at the micro and mesoscale in these materials result in mechanical couplings at the engineering scale (thermal/electrical/dissipative/...) and novel function (e.g., the shape memory effect, piezoelectricity in select metal alloys, the immense fracture toughness of Nacre and like materials, ...). Given this richness in behaviors, my research broadly aims to address the following questions: What happens when active and architectured materials are incorporated into a thin and slender structures? Do phenomena inherent to these materials compete with or enhance those inherent to these structures? Does this interplay result in entirely new and unexpected phenomena? And can all this be exploited to design new functionality in engineering systems?
In this talk, I will explore these questions in the context of thin sheets of an active material in nematic elastomer as well as architectured sheets designed to fold continuously as origami. For the latter, I will completely characterize all rigidly and flat-foldable origami, and describe an effcient algorithm to compute their designs and deformations. For the former, I will show that a material instability inherent to nematic elastomers at the micron scale is capable of suppressing a structural instability (wrinkling) at the engineering scale. These results provide novel, yet concrete, design guidance for improving the effciency solar sails and the performance of other membrane structures (where wrinkling can be an impediment to their functionality), as well as tools to effciently investigate robust and elegant concepts for deployable space structures, reconfigurable antennas, and soft robotics using origami.
Maziar Hemati is a postdoctoral researcher studying the mechanics of Origami, helical structures and shape memory alloys at the University of Minnesota. He attended University of Michigan, receiving a Bachelors of Science in Civil Engineering (2010) and and Masters of Science in Structural Engineering (2011). He then moved to Caltech, where he received a Ph.D in Mechanical Engineering (2017) studying the deformations of thin nematic elastomer sheets. When not folding paper—and when his Achilles is functioning properly—you can often find him on the basketball court.
Wednesday, January 23, 2019 3:30 PM Seaver Science Library, Room 150 (SSL 150)
Refreshments will be served at 3:15 pm.
host: Oberai
Planning and Decision-Making for Energy Efficient and Sustainable Autonomous Systems
Ran Dai
Netjets Assistant Professor Mechanical and Aerospace Engineering Department The Ohio State University Columbus, OH
Many autonomous systems will benefit from prolonged operational time and reduced power consumption in a variety of long-duration missions, ranging from terrestrial operating domain to interplanetary space exploration. Due to limited power capacity, dynamic operating environments, complex system behaviors, and strict mission constraints, it is challenging to realize full autonomy with capabilities of sustained power supply and energy efficient operations. Without human intervention, real-time planning and decision-making, including both motion planning and logic/reasoning decisions, play a critical role in assuring the reliability and performance of such systems toward accomplishing the mission objectives.
This talk will present our work on developing vision-based energy awareness, sophisticated modeling approach, highly implementable optimization algorithms, and machine learning based auto-tuning method that collectively contribute to advanced planning and decision-making strategies for energy efficient and sustainable autonomous systems. Applications in two types of autonomous systems will be discussed. One is solar-powered ground robot that harvests energy from the environment and charges the storage batteries as backup to extend the endurance time or realize persistent operations. The other type of application focuses on space vehicles in complex missions involving multiphase or hybrid operations where onboard propellant is limited and timely ground support is unavailable. The overall objective of real-time planning and decision-making for both types of autonomous systems is to realize high-level autonomy in energy harvesting and utilization under dynamic environments, complex operations, and mission constraints. Results obtained in virtual simulations are verified in real-world environments or experimental platforms that mimic the mission challenges, leading to a synthesized theoretical and experimental framework for evaluating improved performance of this transformational technique.
Ran Dai is a Netjets Assistant Professor in Mechanical and Aerospace Engineering Department at The Ohio State University. She received her B.S. degree in Automation Science from Beihang University and her M.S. and Ph.D. degrees in Aerospace Engineering from Auburn University. After graduation, she worked as an engineer in an automotive technology company, Dynamic Research, Inc., and conducted research and consulting in the areas of semi-autonomous vehicle guidance and control. From 2010 to 2012, Dr. Dai joined the Robotics, Aerospace, and Information Networks Lab at University of Washington as a postdoctoral fellow, where she involved in an energy management project with application to the next generation of Boeing 787 aircraft power systems. Dr. Dai’s research focuses on control and optimization of autonomous systems, motion planning and estimation of space vehicles, and networked dynamical systems. She is a recipient of the National Science Foundation Career Award and NASA Early Faculty Career Award.
Wednesday, February 6, 2019 3:30 PM Seaver Science Library, Room 150 (SSL 150)
Refreshments will be served at 3:15 pm.
host: Flashner
Extremely Agile and Robust Legged Robots
Quan Nguyen
Postdoctoral Associate Biomimetic Robotics Lab Massachusetts Institute of Technology Cambridge, MA
The mobility of man-made machines is still limited to relatively flat grounds, whereas humans and animals can traverse almost all surfaces of the earth including rocky cliffs or collapsed buildings.
In this talk, I will pose the question "How can we make robots with similar morphologies achieve such extremely agile and robust behaviors?" Enabling robots to exhibit such behaviors will one day facilitate robotic space exploration, disaster response, construction, etc. Furthermore, such time and safety critical missions also require robots to operate swiftly and stably while dealing with high levels of uncertainty and large external disturbances.
To achieve these capabilities, a unified adaptive control framework will be presented, that enables the ability to enforce stability and safety-critical constraints arising from robotic motion tasks under a high level of model uncertainty. Next, I will present novel optimization-based approaches to address the challenge of dynamic robotic walking over randomly generated stepping stones, and optimized jumping on high platforms. I will then show how these can be translated to real-world experiments, that enables (a) ATRIAS, a bipedal robot at CMU, to walk dynamically on stepping stones, and (b) MIT's Cheetah 3 robot to jump up onto and jump down from a desk.
Quan Nguyen is a postdoctoral associate at the Biomimetic Robotics Lab, Massachusetts Institute of Technology (MIT). Prior to this, he completed his Ph.D. from Carnegie Mellon University (CMU) in 3.5 years with the Best Dissertation Award. His research interests span different control and optimization approaches for highly dynamic robotics including nonlinear control, trajectory optimization, real-time optimization-based control, robust and adaptive control. His work on the bipedal robot ATRIAS walking on stepping stones was featured on the IEEE Spectrum, TechCrunch, TechXplore and Digital Trends. His work on the MIT Cheetah 3 robot leaping on a desk was featured widely in many major media channels, including CNN, BBC, NBC, ABC, etc. Nguyen won the Best Presentation of the Session at the 2016 American Control Conference (ACC) and the Best System Paper Finalist at the 2017 Robotics: Science & Systems conference (RSS).
Wednesday, February 13, 2019 3:30 PM Seaver Science Library, Room 150 (SSL 150)
Refreshments will be served at 3:15 pm.
host: Flashner
Manufacturability-Driven, Multi-Component Topology Optimization (MTO) for Top-Down Design of Structural Assemblies
Kazuhiro Saitou
Professor Department of Mechanical Engineering University of Michigan Ann Arbor, MI
This talk presents a manufacturability-driven, multi-component topology optimization (MTO) framework for simultaneous design and partitioning of structures assembled of multiple components. Constraints on component geometry imposed by chosen manufacturing processes are incorporated in the conventional density-based topology optimization, with additional design variables specifying fractional component membership which enables continuous relaxation of otherwise discrete partitioning problems. The geometric constraints imposed by various manufacturing processes, such as size, perimeter length, undercut, and enclosed cavities, are also relaxed to enable the manufacturability evaluation of “gray” geometries that occur during the density-based topology optimization. Examples on minimum compliance structural assembly design for sheet metal stamping (MTO-S), die casting (MTO-D), additive manufacturing (MTO-A), and tailored-fiber composite process (MTO-C) show promising advantages over the conventional monolithic topology optimization. In particular, manufacturing constraints previously applied to monolithic topology optimization gain new interpretations when applied to multi-component assemblies, which can unlock richer design space for topology exploration. The talk will conclude with a brief overview of the latest developments on the MTO framework for continuous fiber printing processes and for “4D” printing processes.
Kazuhiro Saitou is Professor of Mechanical Engineering at the University of Michigan, Ann Arbor. He received BEng degree from University of Tokyo, and MS and PhD degrees from the Massachusetts Institute of Technology (MIT), all in Mechanical Engineering. His research interest includes algorithmic and computational design synthesis and design for manufacture and assembly, with applications in mechanical, industrial, and biomedical systems. Prof. Saitou was the recipient of several awards including Kos-Ishii Toshiba Award from ASME Design for Manufacturing and the Life Cycle Technical Committee, Innovative Design Component Award from ARPA-E Lightweighting Technologies Enabling Comprehensive Automotive Redesign (LITECAR) Challenge, Design and Systems Division Achievement Award from Japan Society of Mechanical Engineers, CAREER Award from US National Science Foundation, and best paper awards at international conferences. He has served as an Associate Editor for ASME Journal of Computing and Information Science in Engineering (JCISE), ASME Journal of Mechanical Design (JMD), and IEEE Transactions on Automation Science and Engineering (T-ASE), and is currently serving as an Associate Editor for JCISE (again) and a Senior Editor for T-ASE. He was a past Chair of the ASME Design Automation Technical Committee (DAC) and the ASME Design for Manufacturing and the Life Cycle Technical Committee (DFMLC), and the Editor-In-Chief for Conference Editorial Board of IEEE Conference on Automation Science and Engineering. He is Fellow of ASME and IEEE.
Wednesday, February 20, 2019 3:30 PM Seaver Science Library, Room 150 (SSL 150)
Refreshments will be served at 3:15 pm.
host: Gupta
Smart and Advanced Manufacturing Innovation – Are we living up to the potential?
Sudarsan Rachuri
Technology Manager US Department of Energy
Smart manufacturing is an emerging field that combines physical and intelligence. It can mean different things to different people, but essentially it is about smartly extracting information from the manufacturing system to improve overall efficiency of networked enterprises. Smart manufacturing provides effective and secure human-system platforms for better decision making and improving the overall productivity and efficiency of manufacturing across the networked enterprise. The talk will also describe the future needs of smart manufacturing with respect to disruptive technologies, industry best practices, and, more importantly, workforce and skills development.
Sudarsan Rachuri is a Technology Manager in the Advanced Manufacturing Office, EERE, and DOE. He is the Federal Program Manager for the CESMII. Prior to joining DOE, he was the program manager at National Institute of Standards and Technology (NIST) and also a research professor at George Washington University and worked in the CAD/CAE/PLM software industry.
Dr. Rachuri is the Editor-in-Chief of ASTM Smart and Sustainable Manufacturing Systems journal (www.astm.org/ssms). Rachuri is the founding member and was the vice chair of the ASTM subcommittee on sustainable manufacturing (E60.13) and a member of ASTM Smart Manufacturing Advisory Committee. Rachuri is the founding member and the Chair of the standards committee on ASME V&V 50 Verification and Validation of Computational Modeling for Advanced Manufacturing. Dr. Rachuri was a member of many ISO and ASME standards committees.
Dr. Rachuri is a Fellow of ASME and AAAS (American Association for the Advancement of Science). Dr. Rachuri received the 2016 ASTM International President’s Leadership Award. Dr. Rachuri won first prize in the 2017 World Standards Day (WSD) Paper Competition, awarded by The Society for Standards Professionals. Dr. Sudarsan Rachuri was recently honored with the Excellence in Research Award by the American Society of Mechanical Engineers (ASME) Computers and Information in Engineering (CIE) Division.
Friday, March 1, 2019 2:00 PM USC Viterbi Center for Advanced Manufacturing, Conference Room 2727 S Flower St, Los Angeles, CA 90007
Advanced Manufacturing Seminar Series
host: Gupta
Mechano-Chemical Phase Transformations: Computational Framework, Machine Learning Studies and Graph Theoretic Analysis
Krishna Garikipati
Professor of Mechanical Engineering, and Mathematics and Director, Michigan Institute for Computational Discovery & Engineering Departments of Mechanical Engineering and Mathematics University of Michigan Ann Arbor, MI
Phase transformations in a wide range of materials---for energy, electronics, structural and other applications---are driven by mechanics in interaction with chemistry. We have developed a general theoretical and computational framework for large scale simulations of these mechano-chemical phenomena. I will begin by presenting our recent work in this sphere, while highlighting some of its more insightful results. In addition to being a platform for investigating mechanically driven phenomena in materials physics, this work is a foundation to explore the potential of recent advances in data-driven modeling. Of interest to us are machine learning advances that may enhance our approaches to solve computational materials physics problems. I will outline the first of several recent studies that we have launched in this spirit. Such combinations of classical high-performance scientific computing and modern data-driven modeling now allow us to access large numbers of states of physical systems. They also motivate the study of mathematical structures for representation, exploration and analysis of systems by using these collections of states. With this perspective, I will offer a view of graph theory that places it in nearly perfect correspondence with properties of stationary and dynamical systems. This has opened up new insights to our earlier, large-scale computational investigations of mechano-chemically phase transforming materials systems. This treatment has potential for eventual decision-making for physical systems that builds on high-fidelity computations.
Krishna Garikipati is a computational scientist whose work draws upon nonlinear physics, applied mathematics and numerical methods. A very recent interest of his is the development of methods for data-driven computational science. He has worked for quite a few years in mathematical biology, biophysics and materials physics. Some specific problems he has been thinking about recently are: (1) mathematical models of patterning and morphogenesis in developmental biology, (2) mathematical and physical modeling of tumor growth, and (3) mechano-chemically driven phenomena in materials, such as phase transformations and stress-influenced mass transport.
Wednesday, March 6, 2019 3:30 PM Seaver Science Library, Room 150 (SSL 150)
Refreshments will be served at 3:15 pm.
host: Oberai
Microstructural Engineering Of Energy-Related Materials
Ananya Renuka Balakrishna
Postdoctoral Researcher Department of Aerospace Engineering and Mechanics College of Science & Engineering University of Minnesota, Twin Cities Minneapolis, MN
Future advances in aerospace engineering depend on developing materials with enhanced properties. For example, the next generation of electric aircrafts will need light-weight low-fatigue materials, high-performance sensing and actuation materials, and high-density energy storage materials. Material properties can be drastically enhanced by tuning the materials’ microstructural features. In my research, I develop and apply phase-field methods to investigate how microstructures form and evolve in materials, and how we can engineer these microstructures to enhance material properties. In this talk, I will present applications of phase-field modeling to two material systems: electro-mechanical (ferroelectrics) and chemo-mechanical (batteries) systems. First, I will show how microstructural engineering of ferroelectric materials generate actuation strains several times greater than piezoceramics in market. Second, I will show that not only the electrodes’ microstructures but also their crystallographic texture can be tailored to enhance battery materials’ mechanical strength. Overall, the phase-field models developed in my research provide a theoretical and computation framework to engineer next generation aerospace materials with enhanced properties and extended lifespans.
Ananya Balakrishna is a postdoctoral researcher at the University of Minnesota investigating microstructures in magnetic and light-interactive materials. She completed her PhD in Solid Mechanics and Materials Engineering at the University of Oxford, before pursuing postdoctoral research at MIT as a Lindemann Postdoctoral fellow. Broadly, her research focuses on developing mathematical models to investigate the links between material microstructures and properties in energy storage and functional materials. Her research on engineering ferroelectric microstructures has been recognized by the Falling Walls London Lab prize, and the British Federation for Women Graduates Award. She has also won other awards including the ASME Best student paper award, and the Felix scholarship for her graduate study.
Wednesday, March 20, 2019 3:30 PM Seaver Science Library, Room 150 (SSL 150)
Refreshments will be served at 3:15 pm.
host: Oberai
Advanced Manufacturing of Unconventional 3D Micro- and Meso-Structures: From Strain-Engineered Growth to Mechanically Guided Assembly
Hangbo Zhao
Postdoctoral Researcher Center for Bio-Integrated Electronics Northwestern University Evanston, IL
The growing availability of methods for three-dimensional (3D) manufacturing methods has implications across diverse areas ranging from energy systems to microelectronics, yet few techniques offer the necessary capabilities in geometric complexity, materials compatibility and design versatility. In this talk, I will discuss two novel manufacturing approaches to creating 3D functional material systems that are not feasible by conventional manufacturing methods: 1) strain-engineered growth of complex 3D carbon nanotube microarchitectures, and 2) mechanically guided 3D assembly of a broad range of functional materials and electronics. I will show how strain-engineered growth of carbon nanotubes, in combination with conformal coatings, enables direct formation of hierarchically structured surfaces with tailorable mechanical and interfacial properties for controlling liquid wetting and adhesion. Next, I will describe novel manufacturing technologies that exploit structural buckling and local twisting to create morphable 3D mesoscale structures in diverse advanced materials, and show how these can be used to make tunable optical metamaterials. I will also outline a microphysiological platform fabricated by mechanically guide assembly for tissue engineering and biomedical research. I will conclude by discussing new opportunities in designing and manufacturing multifunctional, adaptive material systems.
Hangbo Zhao is currently a postdoctoral researcher in the Center for Bio-Integrated Electronics in Prof. John Rogers’ group at Northwestern University, where he works on multifunctional 3D materials systems and bio-integrated electronics for applications in tissue engineering and healthcare. He received his Ph.D. degree in the Department of Mechanical Engineering at MIT in 2017, supervised by Prof. A. John Hart. His Ph.D. thesis focused on developing engineered, hierarchical surfaces for controlling liquid wetting and adhesion. He received his master’s degree also in mechanical engineering at MIT in 2014, supervised by Prof. Carl V. Thompson. He received his bachelor’s degree in precision instruments at Tsinghua University in China in 2011.
Wednesday, March 27, 2019 3:30 PM Seaver Science Library, Room 150 (SSL 150)
Refreshments will be served at 3:15 pm.
host: Gupta
—John Laufer Lecture—
Nonlinear Dynamics with Noise
Bala Balachandran
Minta Martin Professor Department of Mechanical Engineering University of Maryland College Park, MD
Nonlinearity influenced dynamics occurs in a variety of mechanical and structural systems. For operations of many of these systems, noise is often viewed as being undesirable. However, the interplay between noise and nonlinearity in a system can result in significant response changes that can be beneficial to a system’s performance. In this spirit, the work carried out to further our understanding on the constructive use of noise in a nonlinear system to realize noise-enhanced responses, noise-enabled stabilization, and noise-assisted response steering will be discussed. Efforts undertaken with partial control will be discussed. Representative physical systems that will be considered include coupled oscillator arrays at the micro-scale and macro-scale, flexible rotor systems, and pendulum systems. The findings of these studies are expected to be relevant to a variety of different nonlinear, mechanical and structural systems. Some thoughts on future directions in the realm of applied nonlinear dynamics will be presented to close the talk.
Bala Balachandran received his B. Tech (Naval Architecture) from the Indian Institute of Technology, Madras, India, M.S. (Aerospace Engineering) from Virginia Tech, Blacksburg, VA and Ph.D. (Engineering Mechanics) from Virginia Tech. Currently, he is a Minta Martin Professor of Engineering at the University of Maryland, where he has been since 1993. His research interests include nonlinear phenomena, dynamics and vibrations, and control. The publications that he has authored/co-authored include over ninety journal publications, a Wiley textbook entitled "Applied Nonlinear Dynamics: Analytical, Computational, and Experimental Methods" (1995, 2006), a third edition of a textbook entitled "Vibrations" (2019) by Cambridge University Press, and a co-edited Springer book entitled “Delay Differential Equations: Recent Advances and New Directions” (2009). He holds four U.S. patents and one Japan patent, three related to fiber optic sensors and two related to atomic force microscopy. He is a Contributing Editor of the International Journal of Non-Linear Mechanics and the Editor of the ASME Journal of Computational and Nonlinear Dynamics. He is a Fellow of ASME and AIAA.
Wednesday, April 3, 2019
Reception at 12:00 NOON Seminar Immediately Following
The Franklin Suite, Third Floor of Tutor Campus Center
host: Udwadia
Towards Robotic Manipulation – Understanding the World Through Contact
Nima Fazeli
Ph.D. Student Mechanical Engineering Department Massachusetts Institute of Technology Cambridge, MA
Why is robotic manipulation so hard? As humans, we are unrivaled in our ability to dexterously manipulate objects and exhibit complex skills seemingly effortlessly. Recent research in cognitive science suggests that this ability is driven by our internal representations of the physical world, built over a life-time of experience. Our predictive ability is complemented by our senses of sight and touch, intuitive state-estimation, and tactile dexterity. Given the complexity of human reasoning, skill, and hardware, it is not surprising that we have yet to replicate our abilities in robots. In order to bridge this gap, we must develop robotic systems that build their understanding and interpretation of the physical world through contact. Using experiments as tools, these Galilean Robots will distill their experiences into models of the physical world.
In this talk, I will present some of my work spanning the spectrum of analytical to fully data-driven methodologies for model building and inference through contact. I believe that Galilean Robots need to master tools from this spectrum for intelligent and dexterous manipulation. First, I’ll discuss a methodology for the inference of contact forces and system parameters of rigid-bodies systems making and breaking contact. I will then touch on data-augmented contact models for controls as a medium between analytical and data-driven techniques. I will show how a robot can learn the physics of playing Jenga using a hierarchical-learning methodology purely from data. I will conclude the talk by providing perspectives on building Galilean Robotic systems that embody intelligent manipulation.
Nima Fazeli is a PhD student with the Mechanical Engineering Department at MIT, working with Prof. Alberto Rodriguez. His research focuses on enabling intelligent and dexterous robotic manipulation by developing novel tools combining analytical methods, machine learning, and cognition/AI. During his PhD, Nima has developed inference algorithms for robotic systems undergoing frictional contact, performed empirical evaluations of contact models, demonstrated data-augmented contact models for manipulation, and developed a robotic system capable of learning the physics of playing Jenga using a hierarchical learning methodology. Nima received his masters from the University of Maryland at College Park where he spent most of his time developing analytical and data-driven models of the human (and, on occasion, swine) arterial tree together with novel inference algorithms to diagnoses cardiovascular diseases. His research has been supported by the Rohsenow Fellowship and featured in outlets such as CBS, CNN, and the BBC. He looks forward to robots playing and learning alongside his grandchildren.
Thursday, April 4, 2019 11:00 AM The Laufer Library (RRB 208)
Refreshments will be served at 10:45 AM.
host: Flashner
Manufacturing and Device Innovations of Rubbery and Curvy Electronics: Toward a Seamless Integration with Humans
Cunjiang Yu
Bill D. Cook Assistant Professor of Mechanical Engineering Department of Mechanical Engineering University of Houston Houston, TX
While human tissues and organs are mostly soft and curvy; conventional electronics are hard and planar. Seamlessly merging electronics with human is of imminent importance in addressing grant societal challenges in health and joy of living. However, the main challenge lies in the huge mechanical mismatch between the current form of rigid electronics and the soft curvy nature of biology.
In this talk, I will first describe a new form of electronics, namely “rubbery electronics”, with skin-like softness and stretchability, which is constructed based upon elastic rubbery electronic materials. As the core basis of rubbery electronics, rubbery semiconductor has been developed through composite engineering based on commercial available materials and manufactured in a scalable and reliable manner. These manufacturing and device innovations set a foundation to realize fully rubbery electronics, circuits and sensors. In particular, rubbery transistors, logic gates, integrated electronics, sensors, smart skins, implants, neuro devices, and integrated function systems will be demonstrated. In the second part of the talk, I will introduce the invention and development of conformal additive stamp (CAS) printing, a novel, reliable and versatile manufacturing technology for developing 3D curvy electronics. Electronics with 3D curvilinear layouts, especially in the size range from millimeter to centimeter with accuracy of microns, are technically very challenge to build. The major hurdle lies in the lack of a proper manufacturing technology. CAS printing has therefore been developed to solve this long-standing manufacturing challenge. Systematic understanding and extensive employment of CAS printing for various curvy electronics will be presented to illustrate its manufacturing fidelity. Devices such as smart contact lens with integrated sensors and electronics for multiple diagnostic functions will be demonstrated. Soft and curvy electronics have open a new paradigm for personal healthcare, medical diagnosis, biological studies, human-machine interfaces, soft machines, etc.
Cunjiang Yu is currently the Bill D. Cook Assistant Professor of Mechanical Engineering at the University of Houston, with joint appointments in Electrical and Computer Engineering, Materials Science and Engineering, and Biomedical Engineering. He completed his Ph.D. in Mechanical Engineering within three years at Arizona State University in 2010 and was trained as a postdoc at the University of Illinois at Urbana-Champaign before joining University of Houston in 2013. Dr. Yu was a recipient of NSF CAREER Award, ONR Young Investigator Award, MIT Technology Review 35 Top Innovators under the age of 35 - TR35 China, Society of Manufacturing Engineers Outstanding Young Manufacturing Engineer Award, Young Investigator Awards from American Vacuum Society and American Chemical Society, 3M Non-Tenured Faculty Award, and a few research and teaching awards at University of Houston. His recent research has been reported or highlighted by many media outlets, such as Time, Discovery, BBC News, NBC News, Science News, USA Today, etc.
Monday, April 8, 2019 3:30 PM Laufer Library (RRB 208)
Refreshments will be served at 3:15 pm.
host: Gupta
The Blood Microenvironment in Thrombosis and Hemostasis: The Good, Bad and the Sticky
Owen McCarty
Professor & Chair Department of Biomedical Engineering Oregon Health & Science University Portland OR
Hemostatic plug formation upon blood vessel breach is initiated by platelet recruitment, activation and aggregation in concert with thrombin generation and fibrin formation. However, a similar process can also lead to pathological processes including deep vein thrombosis, ischemic stroke, or myocardial infarction, among others. We have developed narrow mechanism-specific agents targeting the intrinsic pathway of coagulation and demonstrated that experimental thrombosis and platelet production in primates is interrupted by selective inhibition of activation of coagulation factor (F)XI by FXIIa. In this seminar, I will present new data on the role of the endothelium in inactivating FXI, as well as studies on whether inhibiting FXI is beneficial in a non-human primate model of sepsis. I will present our first data from our clinical trial on the safety of inhibition of FXI, and plans to test the efficacy of FXI inhibition in dialysis. The understanding of the mechanisms by which the intrinsic pathway of coagulation promotes thrombus formation may support the rationale for the development of selective, safe and effective antithrombotic strategies targeting FXI.
Owen McCarty, a native of Rochester, received his B.S. in Chemical Engineering from SUNY Buffalo, and a Ph.D. degree in Chemical Engineering from Johns Hopkins University, where his research focused on the identification and characterization of tumor cell receptors for blood platelets and leukocytes. He performed his postdoctoral research on platelet cell biology in the Pharmacology Department at the University of Oxford and University of Birmingham, UK in the group of Dr. Steve Watson. Dr. McCarty joined Oregon Health & Science University in 2005, where he holds an appointment as a Professor in the Departments of Biomedical Engineering and Cell, Developmental & Cancer Biology and the Division of Hematology & Medical Oncology in the OHSU School of Medicine. Dr. McCarty serves as the Chair of the Biomedical Engineering Department and a fellow of the American Heart Association.
Wednesday, April 10, 2019 3:30 PM Seaver Science Library, Room 150 (SSL 150)
Refreshments will be served at 3:15 pm.
host: Newton
On-Chip Materials Processing and Cell Characterization
Nicole Hashemi
Associate Professor Department of Mechanical Engineering Iowa State University Ames, IA
Micro/nanoscale technologies are emerging as powerful tools for controlling the interaction between cells and their surroundings for biological studies and biomedical applications. I will discuss two areas of research in our lab.
Fibers have been increasingly used in various biomedical applications since they provide cells with a hydrated 3D microenvironment that mimics the native extracellular matrix. We have employed hydrodynamic focusing and solvent extraction to fabricate highly-structured polymer microfibers in a microfluidic platform. The microfibers are used to investigate growth and differentiation of progenitor cells in vitro. The results indicate that the 3D topography of the fibers supports the adhesion, survival, and differentiation of the progenitor cells. This shows the significance of fibrous scaffolds for cell alignment important in applications such as reconnecting serious nerve injuries and guiding the direction of cell growth as well as regenerating blood vessels, tendons, and muscle tissue.
We have also developed various approaches to merge microscale techniques for cell-based screenings. We have designed and developed an optofluidic biosensor for on-chip characterization of microorganisms. Using hydrodynamic focusing and integrated optics, phytoplankton with a wide range of sizes are successfully characterized by their intrinsic fluorescence and scattered light.
Nicole Hashemi is an Associate Professor in the Department of Mechanical Engineering at Iowa State University. Her research interests are in the areas of microfluidics, materials, and biomedical microdevices. She has been the recipient of the NSF EAPSI Fellowship, NRC Postdoctoral Fellowship, NRL Research Publication Award, Big 12 Faculty Fellowship Award, and 2017 ISU Early Career Engineering Faculty Research Award. She has also been selected as both National Academy of Engineering US Frontiers of Engineering and National Academy of Sciences Kavli Frontiers of Science. Her research was highlighted and featured in BBC, Forbes, Science Friday, Fox News, and CNN. Professor Hashemi has published over 80 journal and peer-reviewed conference articles, and presented several invited keynote, national, and international lectures.
Monday, April 15, 2019 3:30 PM The Laufer Library (RRB 208)
Refreshments will be served at 3:15 pm.
Fall, 2019
Fast Spectral Time-Domain PDE Solvers for Complex Structures: The Fourier-Continuation Method
Oscar P. Bruno
Professor Department of Applied and Computational Mathematics CalTech Pasadena, CA
We present fast spectral solvers for time-domain Partial Differential Equations. Based on a novel Fourier-Continuation (FC) method for the resolution of the Gibbs phenomenon, these methodologies give rise to time-domain solvers for PDEs for general engineering problems and structures. The methods enjoy a number of desirable properties, including spectral time evolution essentially free of pollution or dispersion errors for general PDEs in the time domain, with conditional/unconditional stability for explicit/alternating-direction methods and high order of temporal accuracy. A variety of applications to linear and nonlinear PDE problems will be presented, including the diffusion and wave equations, the Navier-Stokes equations and the elastic wave equation, demonstrating the significant improvements the new algorithms can provide over the accuracy and speed resulting from other approaches.
Oscar Bruno received his Ph.D. degree from the Courant Institute of Mathematical Sciences, New York University. Following graduation, he held a two-year position as Visiting Assistant Professor with the University of Minnesota, and he then joined the faculty of the Georgia Institute of Technology (Georgia Tech), where he served as Assistant and Associate Professor. After a four-year period with Georgia Tech, in 1995 he joined the faculty of the California Institute of Technology (Caltech), where he has served as Professor in the Department of Applied and Computational Mathematics since 1998, and as Executive Officer of that department during 1998-2000. Dr. Bruno's research interests lie in areas of optics, elasticity and electromagnetism, remote sensing and radar, overall electromagnetic and elastic behavior of materials (solids, fluids, composites materials, multiple-scale geometries), and phase transitions. Dr. Bruno has directed 37 graduate students and postdocs during his career, and his research efforts have resulted in the publication of more than 100 refereed articles, and have been acknowledged by his plenary presentations at many international conferences, his service on editorial boards of important scientific journals, including the SIAM Journal of Applied Mathematics, the SIAM Journal on Scientific Computing, and the Proceedings of the Royal Society of London, and his election to honorary societies, most notably the Council for the Society for Industrial and Applied Mathematics. Dr. Bruno is a recipient of the Sigma-Xi faculty award, the Friedrichs Award for an outstanding dissertation in mathematics, a Young Investigator Award from the National Science Foundation. and a Sloan Foundation Fellowship. Dr. Bruno is a SIAM Fellow in the class of 2013, and a National Security NSSEFF Vannevar Bush fellow, in the class of 2016.
Wednesday, August 28, 2019 3:30 PM Stauffer Science Lecture Hall, Room 102 (SLH 102)
Refreshments will be served at 3:15 pm.
host: Pahlevan
Insights into the Nonlinear Evolution of Flame Fronts through Experiments and Models
Basile Radisson
Postdoctoral Scholar Department of Aerospace & Mechanical Engineering USC
Due to the exothermicity of combustion reaction, premixed flames are intrinsically unstable. Consequently, cellular patterns are formed on the flame front resulting in rich and complex dynamics. Combustion involves several hundreds of elementary chemical reactions occurring at a submillimetric interface, rendering a detailed description computationally intractable. Simplified models offer much insight into these complex dynamics. Through asymptotic analysis, the shape of the flame front can be described by a set of poles and their dynamic evolution in the complex plane. Here, we demonstrate for the first time the validity of such model in comparison to experimental flame evolution. A premixed flame propagates in a reactive mixture held between two vertically oriented ceramic glass plates separated by a 5mm gap. By extracting a flame front from this experiment, we demonstrate the feasibility of describing the shape of the front by a set of poles' solutions. The flame front dynamics are well described for approximately ten times the characteristic time of the instability. Beyond this time, the comparison is limited by the sensitivity to initial condition. However, by studying statistical properties of the flame front (here statistics of cell sizes), we demonstrate that the pole description is still valid at long time. Moreover, these statistics satisfy a gamma distribution, characteristic of phenomena for which the elementary interaction rule is of additive nature and which results here from the pole to pole attraction. This analytical prediction of the cell-size distribution could be of great interest for the understanding of turbulent flame dynamics.
We will conclude this presentation by commenting on the role of gravity and the influence of burner walls.
Basile Radisson is a post-doctoral scholar in the Aerospace and Mechanical Engineering department at the University of Southern California since June 2019. Prior to joining USC, Basile received his PhD from IRPHE, Aix-Marseille Université, France, working on flame front dynamics under the supervision of C. Almarcha and B. Denet. Basile's research interests lie in instability phenomena driven by geometric non-linearities. He is currently working on snap instabilities in fast elastic filaments under the supervision of E. Kanso.
Wednesday, September 4, 2019 3:30 PM Stauffer Science Lecture Hall, Room 102 (SLH 102)
Refreshments will be served at 3:15 pm.
host: Kanso
Settling of Cohesive Sediment: Particle-resolved Simulations
Eckart Meiburg
Professor Department of Mechanical Engineering UC Santa Barbara Santa Barbara, CA
We develop a physical and computational model for performing fully coupled, grain-resolving Direct Numerical Simulations of cohesive sediment, based on the Immersed Boundary Method. The model distributes the cohesive forces over a thin shell surrounding each particle, thereby allowing for the spatial and temporal resolution of the cohesive forces during particle-particle interactions.
We test and validate the cohesive force model for binary particle interactions in the Drafting-Kissing-Tumbling (DKT) configuration. Cohesive sediment grains can remain attached to each other during the tumbling phase following the initial collision, thereby giving rise to the formation of flocs. The DKT simulations demonstrate that cohesive particle pairs settle in a preferred orientation, with particles of very different sizes preferentially aligning themselves in the vertical direction, so that the smaller particle is drafted in the wake of the larger one. This preferred orientation of cohesive particle pairs is found to remain influential for much larger simulations of 1,261 polydisperse particles released from rest. These simulations reproduce several earlier experimental observations by other authors, such as the accelerated settling of sand and silt particles due to particle bonding, the stratification of cohesive sediment deposits, and the consolidation process of the deposit. This final phase also shows the build-up of cohesive and direct contact intergranular stresses. The simulations demonstrate that cohesive forces accelerate the overall settling process primarily because smaller grains attach to larger ones and settle in their wakes. An investigation of the energy budget shows that the work of the collision forces substantially modifies the relevant energy conversion processes.
Eckart Meiburg received his Ph.D. from the University of Karlsruhe. After a postdoc at Stanford, he became an assistant professor in applied mathematics at Brown. He then moved to USC as associate then full professor. He later moved to UC Santa Barbara.
Meiburg's research interests are fluid dynamics and transport phenomena, primarily computational fluid dynamics. He uses highly resolved direct numerical simulations to investigate physical mechanisms governing the spatio-temporal evolution of a wide variety of geophysical, porous media, and multiphase flow fields. Some of his current interests are gravity and turbidity currents, Hele-Shaw displacements, double-diffusive phenomena in particle laden flows, and internal bores.
Meiburg has received a Presidential Young Investigator Award, a Humboldt Senior Research Award, and a Senior Gledden Fellowship (Institute of Advanced Studies, University of Western Australia). He is fellow of the American Physical Society and the ASME, was the 2012 Lorenz G. Straub Award Keynote Speaker (Univ. Minn.), gave the Ronald F. Probstein Lecture at MIT in 2018, and was Shimizu Visiting Professor at Stanford University.
Wednesday, September 11, 2017 3:30 PM Stauffer Science Lecture Hall, Room 102 (SLH 102)
Refreshments will be served at 3:15 pm.
host: Bermejo-Moreno
Network-Based Characterization, Modeling, and Control of Fluid Flows
Kunihiko (Sam) Taira
Associate Professor Mechanical and Aerospace Engineering UCLA Los Angeles, CA
The network of interactions among fluid elements and coherent structures gives rise to the amazingly rich dynamics of vortical flows. To describe these interactions, we consider the use of mathematical tools from the emerging field of network science that is comprised of graph theory, dynamical systems, data science, and control theory. In this presentation, we discuss ways to describe unsteady fluid flows with vortical-interaction, modal-interaction, and probability-transition networks. The insights gained from these formulations can be used to characterize, model, and control laminar and turbulent flows. We will also discuss some of the challenges of applying network based techniques to fluid flows and the prospects of addressing them through data-inspired techniques.
Kunihiko (Sam) Taira is an Associate Professor of Mechanical and Aerospace Engineering at UCLA. His research focuses on computational fluid dynamics, flow control, and network science. He received his B.S. degree from the University of Tennessee, and his M.S. and Ph.D. degrees from the California Institute of Technology. He is a recipient of the 2013 U.S. Air Force Office of Scientific Research and 2016 Office of Naval Research Young Investigator Awards.
Wednesday, September 18, 2019 3:30 PM Stauffer Science Lecture Hall, Room 102 (SLH 102)
Refreshments will be served at 3:15 pm.
host: Luhar
Design & Implementation of a 3D-PTV System
Dana Dabiri
Associate Professor William E. Boeing Department of Aeronautics & Astronautics University of Washington Seattle, WA
The dream of experimental fluid dynamicists is to be able to measure complex, three-dimensional turbulent flow fields globally with very high spatial and temporal resolution. While we are still far from fully realizing this dream, significant progress has been made towards this goal during the last three decades. Early quantitative measurement methods using Pitot tubes, Venturi tubes and later measurement methods, such as Hot Wire Anemometry (HWA) and Laser-Doppler Anemometry (LDA), by their nature, were measurement methods that provided instantaneous velocity signals at single-points through time. Early emphasis in turbulence research and its theoretical advancement therefore necessitated a statistical description of turbulent flow fields, which relied heavily upon measurements provided by these single-point measurement techniques. Since the early seventies, the discovery of the existence of three-dimensional coherent structures within turbulent flows using qualitative flow visualization methods (i.e. shadowgraphs, Schlieren systems, dye injection, etc.) has been of significant interest for turbulence researchers. While flow visualization techniques have been around since the days of Prandtl, it is only due to the advent of modern imaging, laser, and data acquisition technology has allowed for qualitative flow visualization to become quantitative. These advents have allowed for the development and advancement of are relatively new measurement technique, Particle Image Velocimetry (PIV) and Particle Tracking Velocimetry (PTV) in two dimensions, and more recently in 3 dimensions. Because of its ability to provide global two/three-dimensional kinematic information as well as its ability to map the evolution of coherent structures through time, PIV/PTV has become a powerful tool in studying, understanding, and modeling fluid flow behavior. In this talk, I will describe the particulars of the 3D Particle Tracking Velocimetry method we have developed and touch on some applications in microflows and LES studies.
Dana Dabiri is Associate Professor at the William E. Boeing Department of Aeronautics & Astronautics at the University of Washington, in Seattle. He received his B.S. in Mechanical Engineering at the University of California, San Diego in 1985; he received his M.S. in Mechanical Engineering at the University of California, Berkeley in 1987; and he received his PhD in Aerospace Engineering at the University of California, San Diego in 1992. He was a Post-doc at Caltech from 1992-1993, and continued at Caltech as a research scientist until the end of 2001. In 2002, he joined the faculty at the William E. Boeing Department of Aeronautics & Astronautics as an Assistant Professor, and was promoted as an Associate Professor in 2009. He serves as an associate editor for the Journal of Visualization since 2009. His work pursues developing novel ways for quantitatively visualizing the movements of fluids. Most recently, Professor Dabiri's research activities have developed novel implementations of 2D and 3D digital particle tracking velocimetry (2DPTV & 3DPTV) system that allows for high-resolution measurements of fluid flows.
Wednesday, September 25, 2019 3:30 PM Stauffer Science Lecture Hall, Room 102 (SLH 102)
Refreshments will be served at 3:15 pm.
host: Pahlevan
Navigating in a Turbulent Environment
Mimi Koehl
Professor Department of Integrative Biology University of California, Berkeley Berkeley, CA
When organisms locomote and interact in nature, they must navigate through complex habitats that vary on many spatial scales, and they are buffeted by turbulent wind or water currents and waves that also vary on a range of spatial and temporal scales. We have been using the microscopic larvae of bottom-dwelling marine animals to study how the interaction between the swimming or crawling by an organism and the turbulent water flow around them determines how they move through the environment. Many bottom-dwelling marine animals produce microscopic larvae that are dispersed to new sites by ambient water currents, and then must land and stay put on surfaces in suitable habitats. Field and laboratory measurements enabled us to quantify the fine-scale, rapidly-changing patterns of water velocity vectors and of chemical cue concentrations near coral reefs and along fouling communities (organisms growing on docks and ships). We also measured the swimming behavior of larvae of reef-dwelling and fouling community animals, and their responses to chemical and mechanical cues. We used these data to design agent-based models of larval behavior. By putting model larvae into our real-world flow and chemical data, which varied on spatial and temporal scales experienced by microscopic larvae, we could explore how different responses by larvae affected their transport and their recruitment into reefs or fouling communities. The most effective strategy for recruitment depends on habitat.
Mimi Koehl, a Professor of the Graduate School in the Department of Integrative Biology at the University of California, Berkeley, earned her Ph.D. in Zoology at Duke University. She studies the physics of how organisms interact with their environments, focusing on how microscopic creatures swim and capture food in turbulent water flow, how organisms glide in turbulent wind, how wave-battered marine organisms avoid being washed away, and how olfactory antennae catch odors from water or air moving around them.
Professor Koehl is a member of the National Academy of Sciences and the American Academy of Arts and Sciences, and is a Fellow of the American Physical Society. Her awards include a MacArthur “genius grant”, a Presidential Young Investigator Award, a Guggenheim Fellowship, the John Martin Award (Association for the Sciences of Limnology and Oceanography, for “for research that created a paradigm shift in an area of aquatic sciences”), the Borelli Award (American Society of Biomechanics, for “outstanding career accomplishment”), the Rachel Carson Award (American Geophysical Union, for "cutting-edge ocean science"), and the Muybridge Award (International Society of Biomechanics “highest honor”).
Wednesday, October 2, 2019 3:30 PM Stauffer Science Lecture Hall, Room 102 (SLH 102)
Refreshments will be served at 3:15 pm.
host: Kanso
Active Particles in Complex Fluids
Gwynn Elfring
Associate Professor Department of Mechanical Engineering and Institute of Applied Mathematics University of British Columbia Vancouver, BC
Active particles are self-driven objects, biological or otherwise, which convert stored or ambient energy into systematic motion. The motion of small active particles in Newtonian fluids has received considerable attention, with interest ranging from phoretic propulsion to biological locomotion, whereas studies on active bodies immersed in complex fluids are comparatively scarce. In this talk I will discuss a theoretical formalism for understanding the motion of active particles in complex fluids and then discuss the effects of viscosity gradients, viscoelasticity and shear-thinning rheology in the context of biological locomotion and the propulsion of colloidal Janus particles.
Gwynn Elfring is an Associate Professor in the Department of Mechanical Engineering and the Institute of Applied Mathematics at the University of British Columbia, and currently a Visiting Associate in the Division of Chemistry and Chemical Engineering at the California Institute of Technology. His group at UBC conducts research on biological locomotion and fluid-body interactions in complex fluids and interfaces. Previously, he completed a Ph.D. at the University of California San Diego under the supervision of Eric Lauga and a postdoctoral fellowship with L. Gary Leal and Todd M. Squires at the University of California Santa Barbara.
Wednesday, October 9, 2019 3:30 PM Stauffer Science Lecture Hall, Room 102 (SLH 102)
Refreshments will be served at 3:15 pm.
host: Kanso
Turbulent Flow Interactions with Complex Topography: From Permeable Walls to Barchan Dunes
Kenneth T. Christensen
Viola D. Hank Professor Department of Aerospace & Mechanical Engineering Department of Civil & Environmental Engineering & Earth Sciences University of Notre Dame Notre Dame, IN
This talk will highlight on-going studies of interactions between turbulence and complex topography. The latter is encountered in a multitude of engineering and natural flow scenarios and can lead to significant modifications of the overlying flow as well as unique coupling between the flow and the topography. This talk will focus on two specific flow-topography situations that are often observed in nature: flow overlying a permeable boundary (like a gravel river bed, for example) and flow over barchan dunes – crescent-shaped bedforms that form in aeolian and sub-aqueous environments with a limited sediment supply and relatively unidirectional flow. In the study of permeable boundaries, models were formed from cubic and body-centered arrangements of spheres wherein the surface exposed to the flow was either smooth or rough (the former meaning no spheres protruded into the flow, though the boundary was still permeable). For the barchan-dune work, fixed-bed barchan models were developed to reflect morphologies identified in nature when these dunes come in close proximity. In both cases, the models were fabricated in acrylic and deployed in a refractive-index-matched (RIM) flow facility whose working fluid has the same optical refractive index as the models. The optically-unimpeded access afforded by the RIM methodology, coupled with particle-image velocimetry measurements, provides unique views of the rich flow dynamics associated with both of these turbulence–complex topography interactions.
Kenneth T. Christensen is the Viola D. Hank Professor at the University of Notre Dame, with a joint appointment in the Departments of Aerospace & Mechanical Engineering and Civil & Environmental Engineering & Earth Sciences. He also presently serves as the Department Chair of Aerospace & Mechanical Engineering and recently completed a two-year term as a Provost Fellow at Notre Dame. He joined the ND faculty after ten-plus years on the faculty at the University of Illinois at Urbana-Champaign. Christensen directs a research group that pursues experimental studies of turbulence, geophysical flows and microfluidics and is a WPI Principal Investigator in the Carbon Dioxide Storage Division of the International Institute for Carbon-Neutral Energy Research (I2CNER) based at Kyushu University in Japan. He is a Fellow of AAAS, APS and ASME, an Associate Fellow of AIAA and has received the AFOSR Young Investigator Award (2006), the NSF CAREER Award (2007), the Francois Frenkiel Award for Fluid Mechanics from APS-DFD (2011) and the Gustus Larson Memorial Award from ASME (2016).
Wednesday, October 16, 2019 3:30 PM Stauffer Science Lecture Hall, Room 102 (SLH 102)
Refreshments will be served at 3:15 pm.
host: Pantano
Neural-Inspired Sparse Sensing and Control for Agile Flight
Bing Brunton
Professor
Winged insects perform remarkable aerial feats in uncertain, complex fluid environments. This ability is enabled by sensation of mechanical forces to inform rapid corrections in body orientation. Curiously, machanoreceptor neurons do not faithfully report forces; instead, they are activated by specific time-histories of forcing. In a set of results that combine biomechanical modeling, sparse sensing mathematical theory, and neural encoding, we find that, far from being a bug, neural encoding by biological sensors is a feature that acts as built-in temporal filtering superbly matched to detect body rotation. Indeed, this encoding further enables surprisingly efficient detection using only a small handful of neurons at key locations. Our ongoing and future work explores biological strategies to use smart data as an alternative to big data, including understanding and designing neural-inspired sensors and actuator to achieve hyperefficient sensing and control for agile flight.
Thursday, October 17, 2019 11:00 AM Biegler Hall, Room 112 (BHE 112)
host: Kanso
Aortic Stiffness, Pressure and Flow Pulsatility and Cardiovascular Disease
Gary F. Mitchell, MD
President Cardiovascular Engineering, Inc. Norwood, MA
The aorta provides a critical buffer between the heart and peripheral organs. Juxtaposition of the highly compliant aorta with stiff conduit arteries creates impedance mismatch and local wave reflection that limits transmission of potentially harmful pulsatile energy into the fragile microcirculation. Aortic wall stiffness increases progressively throughout the human lifespan. However, from young adulthood through midlife, pressure pulsatility actually falls as a result of aortic remodeling to a larger lumen area, which reduces characteristic impedance of the aorta. From midlife onward, aortic wall stiffening accelerates and characteristic impedance and pressure pulsatility increase markedly and contribute to the epidemic of wide pulse pressure (isolated systolic) hypertension. After this midlife transition, stiffness of the aorta exceeds that of the muscular arteries, leading to impedance matching and diminished wave reflection, which increases transmission of excessive pressure and flow pulsatility into the microcirculation. Excessive pulsatility in the microcirculation, particularly in high flow organs such as the brain and kidneys, causes microvascular damage, remodeling and dysfunction, leading target organ damage. In addition, aortic stiffening adds to load on the heart and may interfere with diastolic function, adding to risk for heart failure in late life. Successful interruption of the unfavorable aortic stiffening cascade likely will require early intervention and lifelong prevention, although attempts to reverse the process have been inadequately examined at present.
Gary F. Mitchell is a cardiologist and internationally acknowledged leader in the field of vascular stiffness and pulsatile hemodynamics. He received his medical degree from Washington University in St. Louis and completed his training in Medicine and Cardiology at Brigham and Women’s Hospital in Boston, where he served as a staff cardiologist until 1998. He left the Brigham in 1998 to become founder and president of Cardiovascular Engineering, Inc., which is an NIH-funded small business that designs and develops innovative devices that measure arterial stiffness. Dr. Mitchell and colleagues have used those devices to examine genetic and environmental correlates of arterial stiffness and the role that arterial stiffness plays in the pathogenesis of hypertension and target organ damage. He joined the Framingham Heart Study as a Framingham Investigator in 1999 and became a collaborator on the AGES-Reykjavik study in 2006 and the Jackson Heart Study in 2010. Using devices designed and built by Cardiovascular Engineering, Dr. Mitchell has performed detailed assessments of arterial stiffness and pulsatile hemodynamics in more than 20,000 research participants, including participants in all 3 generations of the Framingham Heart Study as well as participants in the AGES-Reykjavik study, the REFINE study and the Jackson Heart Study.
Wednesday, October 23, 2019 3:30 PM Stauffer Science Lecture Hall, Room 102 (SLH 102)
Refreshments will be served at 3:15 pm.
host: Pahlevan
From Beating Hearts to Buzzing Wings: Flow Physics and Computation at the Intersection of Mechanics and Bioengineering
Rajat Mittal
Professor Department of Mechanical Engineering Johns Hopkins University Baltimore, Maryland
The unceasing growth in computational power and the development of new software tools and numerical algorithms is opening up exciting areas of research, discovery & translation in mechanics and biomedical engineering. Consider the mammalian heart, which has been sculpted by millions of years of evolution into a flow pump par excellence. During the typical lifetime of a human, the heart will beat over three billion times and pump enough blood to fill over sixty Olympic-sized swimming pools. Each of these billions of cardiac cycles is itself a manifestation of a complex and elegant interplay between several distinct physical domains including electrophysiology, muscle mechanics, hemodynamics, flow-induced valves dynamics, acoustics, and biochemistry. Computational models provide the ability to explore such multi-physics problems with unprecedented fidelity and precision. In my talk, I will describe several projects that demonstrate the application of powerful computational tools to problems ranging from chemo-fluidics of clot formation to fluid-structure interaction in prosthetic heart valves. The talk will culminate with a brief discussion on a new project where we are using computational aeroacoustics to analyze wing-tone based communication in mosquitoes.
Rajat Mittal is Professor of Mechanical Engineering at the Johns Hopkins University (JHU) with a secondary appointment in the School of Medicine. He received the B. Tech. degree from the Indian Institute of Technology at Kanpur in 1989, and the Ph.D. degree in Applied Mechanics from The University of Illinois at Urbana-Champaign, in 1995. His research interests include fluid mechanics, computing, biomedical engineering, biofluids and flow control. He is the recipient of the 1996 Francois Frenkiel Award from the Division of Fluid Dynamics of the American Physical Society, and the 2006 Lewis Moody Award from the American Society of Mechanical Engineers. He is a Fellow of American Society of Mechanical Engineers and the American Physical Society, and an Associate Fellow of the American Institute of Aeronautics and Astronautics. He is associate editor of the Journal of Computational Physics, Frontiers of Computational Physiology and Medicine and the Journal of Experimental Biology.
Wednesday, October 30, 2019 3:30 PM Stauffer Science Lecture Hall, Room 102 (SLH 102)
Refreshments will be served at 3:15 pm.
host: Pahlevan
Fabrication, Mechanical Characterization, and Modeling of 3D Architected Materials upon Static and Dynamic Loading
Carlos M. Portela
Postdoctoral Scholar Department of Mechanical and Civil Engineering California Institute of Technology Pasadena, CA
Architected materials have been ubiquitous in nature, enabling unique properties that are unachievable by monolithic, homogeneous materials. Inspired by natural processes, man-made three-dimensional (3D) architected materials have been reported to enable novel mechanical properties such as high stiffness-to-density ratios or extreme resilience, increasingly so when nanoscale size effects are present. However, most architected materials have relied on advanced additive manufacturing techniques that are not yet scalable and yield small sample sizes. Additionally, most of these nano-and micro-architected materials have only been studied in the static regime, leaving the dynamic parameter space unexplored.
In this talk, we discuss advances in our understanding of architected materials by: (i) proposing numerical and theoretical tools that predict the behavior of architected materials with non-ideal geometries, (ii) presenting a pathway for scalable fabrication of tunable nano-architected materials, and (iii) exploring the response of nano-and micro-architected materials under three types of dynamic loading. We first explore the mechanics of lattice architectures with features at the micro-and millimeter scales, and discuss the effect of nodes (i.e., junctions) to obtain more accurate computational and theoretical predictive tools. Going beyond lattices, we propose alternative node-less geometries that exhibit extreme mechanical resilience at the nanoscale, and we harness self-assembly processes to demonstrate a pathway to fabricate them in cubic-centimeter volumes while maintaining nanoscale resolution. Lastly, we venture into the dynamic regime by designing, fabricating, and testing micro-architected materials that exhibit vibrational band gaps in the MHz regime as well as nano-architected materials with extreme energy absorption upon microparticle supersonic impact.
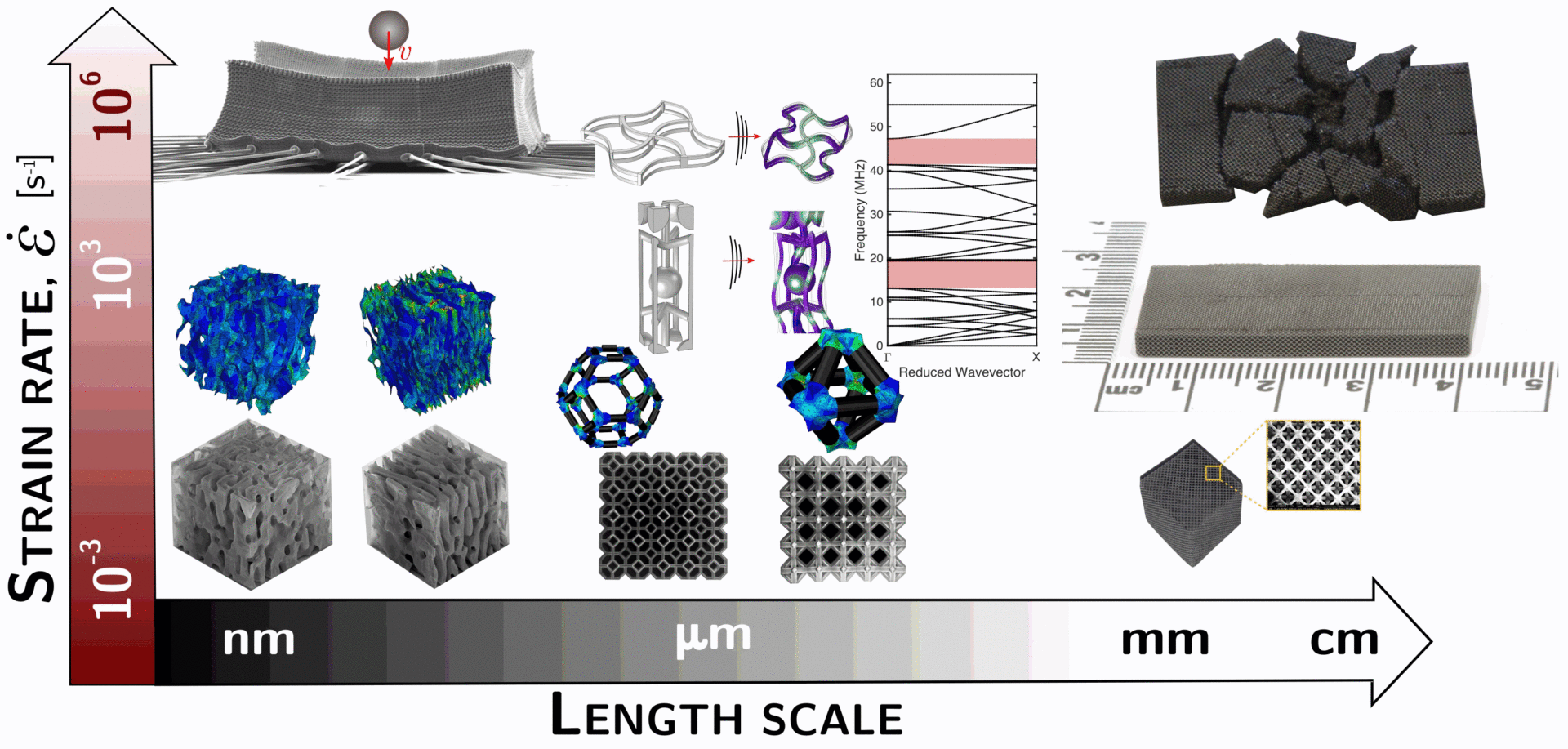
Carlos M. Portela is a postdoctoral scholar in Applied Physics and Materials Science at the California Institute of Technology. He received his Ph.D in mechanical engineering at Caltech, working with Professors Julia R. Greer and Dennis M. Kochmann (ETH Züaut;urich) on the fabrication, testing, and modeling of 3D architected materials with nanometer-to centimeter-scale dimensions. He obtained his M.S. in Mechanical Engineering at Caltech in 2016, and a B.S. in Aerospace Engineering and a B.A. in Physics from the University of Southern California (USC). Carlos is the recipient of the 2019 Gold Paper Award from the Society of Engineering Science (SES), the Centennial Award for the Best Thesis in Mechanical and Civil Engineering at Caltech, and his work has been featured in The National Nanotechnology Initiative Supplement to the President's 2020 Budget (National Science and Technology Council). His research interests lie at the intersection of materials science and mechanical engineering, striving to provide pathways for architected materials to aid in tackling multi-disciplinary challenges.
Wednesday, November 6, 2019 3:30 PM Stauffer Science Lecture Hall, Room 102 (SLH 102)
Refreshments will be served at 3:15 pm.
hosts: Pahlevan & Shiflett
Two Problems in Wall-Bounded Flow: Fluid Energy Extraction in Wind Farms, and Surfactant Effects in Superhydrophobic Drag Reduction
Paolo Luzzatto-Fegiz
Assistant Professor Department of Mechanical Engineering University of California, Santa Barbara Santa Barbara, CA
In this talk, we consider two fluid problems directly linked to decarbonization efforts. In the first part, we investigate fundamental limits to the performance of large wind farms. Since wind turbines are often deployed in arrays of hundreds of units, wake interactions can lead to drastic losses in power output. Remarkably, while the theoretical “Betz” maximum has long been established for the output of a single turbine, no corresponding theory appears to exist for a generic, large-scale energy extraction system. We develop a model for an array of energy-extracting devices of arbitrary design and layout, first focusing on the fully-developed regime, which is relevant for large wind farms. We validate our model against data from field measurements, experiments and simulations. By defining a suitable ideal limit, we establish an upper bound on the performance of a large wind farm. This is an order of magnitude larger than the output of existing arrays, thus supporting the notion that large performance improvements may be possible.
In the second part of this talk, we examine flow past superhydrophobic surfaces (SHS). These coatings have long promised large drag reductions; however, experiments have provided inconsistent results, with many textures yielding little or no benefit. By performing surfactant-laden simulations and unsteadily-driven experiments, we demonstrate that surfactant-induced Marangoni stresses can be to blame. We find that extremely low surfactant concentrations, unavoidable in practice, can drastically increase drag, at least in laminar flows. To obtain accurate drag predictions on SHS, one must therefore solve the mass, momentum, bulk surfactant and interfacial surfactant conservation equations, which is not feasible in most applications. To address this issue, we propose a theory that captures how the near-surface dynamics depend on the seven dimensionless groups for surfactant. We validate our theory extensively in 2D, and describe progress toward 3D and turbulent models. Our theory significantly improves predictions relative to a surfactant-free one, which can otherwise overestimate drag reduction by several orders of magnitude.
Here are links to papers/resources that form the basis for this talk:
- https://doi.org/10.1103/PhysRevFluids.3.093802
- https://doi.org/10.1073/pnas.1702469114
- https://doi.org/10.1103/PhysRevFluids.3.100507
- https://doi.org/10.1103/APS.DFD.2017.GFM.V0098
- https://arxiv.org/abs/1904.01194
Paolo Luzzatto-Fegiz graduated with a BEng in Aerospace Engineering from the University of Southampton, where he received the Royal Aeronautical Society Prize for highest first-class degree and the Graham Prize for best experimental project in the School of Engineering Sciences. After a summer working with the ATLAS Magnet Team at CERN, he completed an MSc in Applied Mathematics at Imperial College, and an MS and PhD in Aerospace Engineering at Cornell University. His doctoral work received the Acrivos Award of the American Physical Society for outstanding dissertation in Fluid Dynamics at a U.S. university. He was awarded a Devonshire Postdoctoral Scholarship from the Woods Hole Oceanographic Institution, as well as a Junior Research Fellowship from Churchill College, Cambridge. He is currently an Assistant Professor in Mechanical Engineering at UCSB, where he has received the Northrop Grumman Teaching Award and a Gallery of Fluid Motion Award from APS-DFD. He co-invented a salinity sensor for oceanography that has been adopted by 20 institutions, and led the first microgravity experiment from NSF CBET, which successfully returned in January 2019 from the International Space Station.
Wednesday, November 13, 2019 3:30 PM Stauffer Science Lecture Hall, Room 102 (SLH 102)
Refreshments will be served at 3:15 pm.
host: Luhar
Closed Nonorientable Ribbons from Unstretchable Helicoidal Material Surfaces
Eliot Fried
Professor Mathematics, Mechanics, and Materials Unit Okinawa Institute of Science and Technology Graduate University(OIST) Okinawa, Japan
A material surface is unstretchable as a two-dimensional physical object if the intrinsic length between each pair of its material points cannot change during any deformation. Intuitively, such a surface can bend and twist but its material filaments can never extend or contract. The constraint that models this intense kinematic idealization must affirm that no surface strain can be developed in any possible deformation, and it must allow for the existence of constraint reactions and the consequential development of related tractions in any deformation. This talk will focus on a theory for determining the shape of closed ribbons made from bending and twisting a unstretchable helicoidal material surface. Surprising connections to the kinematics of underconstrained linkages, the dynamics of closed vortex filaments, and the chemistry of cyclic hydocarbon compounds will be discussed.
Eliot Fried earned his Ph.D. in Applied Mechanics from the California Institute of Technology in 1991. He received a National Science Foundation Mathematical Sciences Postdoctoral Fellowship, a Japan Society for the Promotion of Science Postdoctoral Research Fellowship, and a National Science Foundation Research Initiation Award. Currently he heads the Mathematics, Mechanics, and Materials Unit. Previously, at McGill University, he was a Professor of Mechanical Engineering and the Tier 1 Canada Research Chair in Interfacial and Defect Mechanics. Before that he held tenured positions in the Department of Theoretical and Applied Mechanics at the University of Illinois at Urbana-Champaign and the Department of Mechanical Engineering & Materials Science at Washington University in St. Louis. At Illinois, he was a Fellow of the Center of Advanced Study and was awarded a Critical Research Initiative Grant. In his research, he uses statistical and continuum mechanics and thermodynamics, geometry, asymptotic analysis, bifurcation theory, and scientific computing to study fundamental and applied problems involving novel material systems and processes.
Wednesday, November 20, 2019 3:30 PM Stauffer Science Lecture Hall, Room 102 (SLH 102)
Refreshments will be served at 3:15 pm.
host: Oberai
Patient-Secific Modeling for Virtual Treatment Planning in Pediatric Cardiology
Alison Marsden
Associate Professor Departments of Pediatrics and Bioengineering, and by courtesy of Mechanical Engineering Stanford University Stanford, CA
Cardiovascular disease is the leading cause of death worldwide, with nearly 1 in 4 deaths caused by heart disease alone. In children, congenital heart disease affects 1 in 100 infants, and is the leading cause of infant mortality in the US. Patient-specific modeling based on medical image data increasingly enables personalized medicine and individualized treatment planning in cardiovascular disease patients, providing key links between the mechanical environment and subsequent disease progression. We will discuss recent methodological advances in cardiovascular simulations, including (1) optimization and uncertainty quantification for surgical planning, and (2) a unified finite element formulation for fluid structure interaction towards fluid solid growth. Clinical application of these methods will be demonstrated in two applications: 1) a novel surgical method for stage one single ventricle palliation, and 2) virtual treatment planning in pediatric patients with peripheral pulmonary stenosis. We will also provide an overview of our open source SimVascular project, which makes our tools available to the scientific community (www.simvascular.org). Finally, we will provide an outlook on recent successes and challenges of translating modeling tools to the clinic.
Alison Marsden is an associate professor in the departments of Pediatrics, Bioengineering, and, by courtesy, Mechanical Engineering at Stanford University. She is a member of the Institute for Mathematical and Computational Engineering. From 2007-2015 she was a faculty member in Mechanical and Aerospace Engineering at UCSD. She graduated with a BSE degree in Mechanical Engineering from Princeton University in 1998, and a PhD in Mechanical Engineering from Stanford in 2005. She was a postdoctoral fellow at Stanford University in Bioengineering from 2005-07. She was the recipient of a Burroughs Wellcome Fund Career Award at the Scientific Interface in 2007, an NSF CAREER award in 2011, and was elected as a fellow of AIMBE and SIAM in 2018. She has published over 100 peer reviewed journal papers and serves on the editorial board of several journals. Her research focuses on the development of numerical methods for cardiovascular blood flow simulation and application of engineering tools to impact patient care in cardiovascular surgery and congenital heart disease.
Wednesday, December 4, 2019 3:30 PM Stauffer Science Lecture Hall, Room 102 (SLH 102)
Refreshments will be served at 3:15 pm.
host: Bermejo Moreno