The Search for Stable Nanostructured Materials
Andrea M. Hodge
Assistant Professor
Department of Aerospace & Mechanical Engineering
University of Southern California
Los Angeles, CA 90089
Highly nanotwinned (nt) metals have shown a strength comparable to nanocrystalline metals, while maintaining other desired properties including ductility, conductivity, and thermal stability. However, the deformation mechanisms and mechanical stability of the nt metals is not yet fully understood. Therefore in this presentation, results from highly aligned nt-Cu samples tested in compression, torsion, and tension under various loading/testing conditions relative to the twin boundary (TB) direction (represented in Fig. 1) will be presented. The microstructures of the tested samples were analyzed before and after deformation for each loading configuration in order to study the stability of the nanotwins.
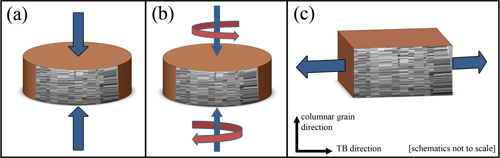
Figure 1: Schematic of the testing configurations used in this study. (a) compression; (b) high pressure torsion (HPT); and (c) tension. Note the direction of loading relative to the twin boundary (TB) and columnar grain directions.
In all testing configurations, the nt structure was observed to be mostly stable, in which, to a significant extent, the nanotwins survived without major changes in twin size, orientation, or twin density. However, distinct differences in the overall deformation of the samples and in the extent of the changes were observed. Elevated temperature stability will also be dicussed.
Wednesday, January 18, 2012
3:30 PM
Seaver Science Library, Room 150 (SSL 150)
Refreshments will be served at 3:15 pm.
On a Few Puzzles in Interfacial Fluid Mechanics
Rouslan Krechetnikov
University of California at Santa Barbara
Santa Barbara, CA
Interfacial fluid mechanics has always been an inexhaustible source of challenging questions. In this talk I will discuss three long-standing problems and our recent progress on their understanding. First, I will present the experiments on self-agitation of a pendant drop, which results from a chemical reaction at the drop interface and which generates a number of nontrivial features: nonlinear auto-oscillations, tip-streaming and droplet trajectory splitting. While some of these features are analogous to the ones in the Taylor cone problem, preliminary analysis revealed that the underlying physics is fundamentally different. The second problem addresses the controversy in the current understanding of Marangoni effects in the classical Landau-Levich problem of a film deposition by a substrate withdrawal. The talk concludes with a discussion of the nature of the crown forming instability in the drop splash problem. Namely, I will describe some recent experiments on the drop splash problem, in which a drop impinges on a thin film of the same liquid, and provide some novel theoretical insights into the nature of the fundamental instability responsible for the crown formation.
Wednesday, January 25, 2012
3:30 PM
Seaver Science Library, Room 150 (SSL 150)
Refreshments will be served at 3:15 pm.
Multiscale Modeling of Phase Transition in Superelastic Nitinol Polycrystals
Panos Papadopoulos
Professor
Department of Mechanical Engineering
University of California, Berkeley
Berkeley, CA 94720-1740
Nitinol is a Ni-Ti alloy used widely in biomedical devices, such as endovascular stents, angioplasty guidewires, vena-cava filters, etc.. One of the major attractions of Nitinol as a biomedical material is its superelastic behavior. This is due to a stress-induced solid- solid phase transformation which accommodates large deformations that may be completely reversed upon removal of the stress. Most constitutive models of phase transformation in superelastic Nitinol account for the austenite-martensite transformation only, and disregard the formation of an intermediate rhombohedral phase (R-phase). However, the presence of R-phase influences the formation of martensitic variants, thus rendering the transformation process path-dependent. In this seminar, a path-dependent micromechanics-based model that incorporates all possible transformations is presented first for the stress-induced phase transformation of Nitinol single crystals. This is subsequently extended to a multiscale thermomechanical model which accounts for the presence of polycrystalline texture. An efficient numerical strategy for solving the resulting constitutive equations is also proposed within a finite element setting.
Friday, January 27, 2012
11:00 AM
Seaver Science Library, Room 150 (SSL 150)
Multiple Equilibria of Inflatable Structures
Sergio Pellegrino
Graduate Aerospace Laboratories
California Institute of Technology
Pasadena, CA
The shape of inflatable structures is determined by overall energy considerations coupled with the unilateral constraints imposed by their envelope (which is unable to carry compression) and by frictional self-contact. These ingredients lead to the existence of multiple equilibrium configurations, however our everyday experience with inflatable structures leads us to assume uniqueness. We tend to associate alternative equilibrium shapes with clearly anomalous deployment. Thus, the observation of large S-clefts during test flights of NASA superpressure balloons was initially attributed to some of kind of deployment anomaly, as these counterintuitive equilibrium shapes had not been seen before and could not be explained without first understanding the shape of clefted balloons. In this talk I will review some experiments that have confirmed the initial flight test observations and I will present an analysis that captures key aspects of the evolution of clefting during deployment and leads to S-clefts that closely resemble those seen in the experiments.
Wednesday, February 1, 2012
3:30 PM
Seaver Science Library, Room 150 (SSL 150)
Refreshments will be served at 3:15 pm.
A Computational Model of Microtubule-Based Motion in the Single-Celled C. elegans Embryo
Tamar Shinar
Amrik Singh Poonian Assistant Professor
Computer Science & Engineering Department
University of California, Riverside
Riverside, CA
We develop a simple model of microtubule-based pronuclear motion in a single-celled C. elegans embryo. The model consists of a model for microtubule dynamic instability, a Newtonian, viscous fluid contained within an enclosing geometry for the cytoplasm, a rigid body for the pronucleus, and a motor protein load-velocity relationship. Motor proteins distributed throughout the cytoplasm interact with microtubule filaments by sliding along them with a velocity that depends on their load. They in turn pull on the filaments, resulting in translation of the microtubule-bound pronucleus. Our simulations show pronuclear migration, and moreover, a robust pronuclear centration and rotation very similar to that observed in vivo. I will also describe the numerical method for the coupled simulation of the Stokes fluid and rigid structures.
Wednesday, February 8, 2012
3:30 PM
Seaver Science Library, Room 150 (SSL 150)
Refreshments will be served at 3:15 pm.
Cracks, Dynamics & the Piezoelectric Effect
Leslie Lamberson
Postdoctoral Research Scholar
Center for Advanced Metallic and Ceramic Systems
Johns Hopkins University
Baltimore, Maryland
and
Assistant Professor
Mechanical Engineering and Mechanics Department
Drexel University
Philadelphia, PA
While a large amount of data is available on the properties and behavior of piezoelectric ceramics subjected to small strains and electric fields as used in conventional sensor and actuator applications, little data exists which contributes to a basic understanding of the behavior of piezoelectric ceramics subjected to high-rate impulsive loading. This particular loading regime plays a critical role in defense applications, specifically for blast mitigation and ballistic protection since numerous armor ceramics such as silicon carbide and aluminum nitride exhibit piezoelectric properties. In addition, piezoelectric materials are also valued for their ability to be utilized as a single-shot, high-energy power supply (or switch) when pulverized, as well as in energy resource recovery applications. In order to utilize these materials ‘smart’ ability, the frequency-time response plays a crucial role in failure, and depends on both the mechanism of polarization, as well as the effect of damage on polarization.
This talk focuses on high strain rate dynamic electromechanical experiments (103 s-1) conducted on single crystal α-quartz, single crystal silicon carbide and aluminum nitride. The results exhibit unexpected trends stress-charge behavior during damage evolution. Specifically, when quartz is undergoing extensive and irreversible dynamic brittle fracture under a compressive stress impulse of up to 2 GPa, the effective piezoelectric stress coefficient increases from loading to unloading. The experimental results are examined in the framework of the theory of linear piezoelectricity and compared to traditional continuum damage models, in order to understand the role of increasing crack density on electroelastic properties.
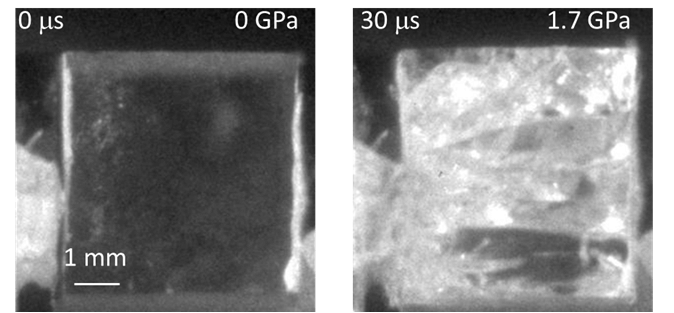
Research sponsored by the Army Research Laboratory.
Dr. Leslie Lamberson has an appointment as an Assistant Professor in the Mechanical Engineering and Mechanics Department at Drexel University, and is presently a Postdoctoral Research Scholar at the Center for Advanced Metallic and Ceramic Systems at the Johns Hopkins University. Her research encompasses high strain rate material system behavior. Leslie received her BS in Aerospace Engineering from the University of Michigan, and MS in the same discipline from the Georgia Institute of Technology. Working with Professor Ares Rosakis, she completed her Ph.D. in Aeronautics from the California Institute of Technology examining hypervelocity impact induced dynamic fracture behavior of brittle polymers.
Wednesday, February 15, 2012
3:30 PM
Seaver Science Library, Room 150 (SSL 150)
Refreshments will be served at 3:15 pm.
Grain Boundary Networks: From Consideration of the Individual Constituents to the Collective Response
Mukul Kumar
Staff Scientist
Physical & Life Sciences Directorate
Lawrence Livermore National Laboratory
Livermore, CA 94550
It has been demonstrated that mechanical response, particularly environmental degradation, of FCC metals and alloys can be improved by exercising control over the population of grain boundary types in the microstructure. The studies also suggest that such properties tend to have percolative mechanisms that depend on the topology of the grain boundary network. Grain boundary engineering investigations have been facilitated by the emergence of SEM-based automated electron backscatter diffraction (EBSD) that enables the characterization of statistically significant datasets of interface crystallography. The EBSD datasets have been analyzed to quantify microstructures in terms of grain boundary character and triple junction distributions. Perhaps more significantly, these large datasets also enable us to visualize crystallographically correlated domains of multiple grains that have been shown to strongly influence crack propagation through the microstructure. Examples from studies on hydrogen and weld embrittlement, stress corrosion cracking, and fatigue will be presented to demonstrate these points along with the constitutive response of such microstructures.
This work performed under the auspices of the U.S. Department of Energy by Lawrence Livermore National Laboratory under Contract DE-AC52-07NA27344.
Mukul Kumar is a Staff Scientist in the Physical & Life Sciences Directorate at LLNL. Prior to joining LLNL, he received his PhD from the University of Cincinnati and had a stint as a postdoctoral fellow at Johns Hopkins University. His research activities have revolved around correlating microstructures with the macroscopic response of the material. This has involved diverse conditions such as travelling strong shock waves to challenging environments seen in jet engines and nuclear reactors. There is growing involvement in taking the next step of formulating predictive models for materials behavior, particularly damage and fracture, and translating them into processing routes for optimized microstructures.
Wednesday, February 22, 2012
3:30 PM
Seaver Science Library, Room 150 (SSL 150)
Refreshments will be served at 3:15 pm.
Source Estimation by Full Wave Form Inversion
Anders Petersson
Center for Applied Scientific Computing
Lawrence Livermore National Laboratory
Livermore, CA
We discuss the inverse problem of determining the source parameters of a small seismic event (location, mechanism, start time, frequency), such that the wave form misfit between seismographic recordings and simulated ground motions is minimized. Our approach is based on direct numerical simulations of the elastic wave equation, allowing for complex heterogeneous material models and realistic topography. A non-linear conjugated gradient approach is applied to solve the inverse problem, where the gradient of the misfit (with respect to the source parameters) is calculated from the numerical solution of an adjoint wave equation. Numerical experiments on simple 2-D models illustrate the importance of scaling the source parameters before applying the conjugated gradient iteration, preferably using the Hessian. A procedure based on arrival times is used to generate an initial guess for the source parameters. For the cases considered here, the conjugate gradient iteration often converges in 20-50 iterations.
Solving the inverse problem requires of the order O(100) numerical solutions of the elastic wave equation. For 3-D models, such problems can only be solved on large parallel machines. We will present the capabilities of our parallel open source code WPP, which was designed to solve seismic wave propagation problems on the regional scale. A higher order accurate scheme is currently being implemented to improve the the frequency resolution and efficiency of the method. These enhancements will be important for solving the three-dimensional inverse problem, for example in geothermal applications where there is interest in using micro seismicity for imaging the geometry of a fractured network.
This work performed under the auspices of the U.S. Department of Energy by Lawrence Livermore National Laboratory under Contract DE-AC52-07NA27344. This is contribution LLNL-ABS-523199.
Wednesday, February 29, 2012
3:30 PM
Seaver Science Library, Room 150 (SSL 150)
Refreshments will be served at 3:15 pm.
Analysis of Multiscale and Multiphysics Problems
Young W. Kwon
Distinguished Professor
Dept. of Mechanical & Aerospace Engineering
Naval Postgraduate School
Monterey, California, USA
ywkwon@nps.edu
In order to better understand the behavior of materials and structures, multiscale and multiphysics analyses are useful and sometimes critical. For example, progressive damage and failure of laminated composite structures can be modeled successfully using the multiscale modeling technique. By doing so, damage and failure in composites can be described in terms of the constituent materials such as fibers and binding matrix regardless of the layer orientation, fiber architecture in each layer, etc. In other words, any damage and failure mode can be described in terms of fiber breakage, matrix cracking, and interface debonding. On the other hand, when a composite structure is in contact with water and subjected to dynamic loading, fluid-structure interaction plays an important role because composite has very comparable density as the water. Therefore, a multiphysics analysis is necessary to understand and predict the structural behavior with fluid-structure interaction. Various multiscale and multiphysics problems are presented.
Wednesday, March 7, 2012
3:30 PM
Seaver Science Library, Room 150 (SSL 150)
Refreshments will be served at 3:15 pm.
Biological Propulsion, Biomimetics, and Flow Control: The Mechanics of Fin Propulsion and a Self-Excited Flapper
Oscar M. Curet
Postdoctoral Fellow
School of Engineering
Brown University
Providence, RI
Swimming and flying animals have a remarkable ability to navigate through complex environments. As they propel themselves, they interact with the surrounding fluid medium. This fluid-animal interaction plays a fundamental factor in how animals actuate their muscle as well as how they have evolved. In order to incorporate biological-based designs into the next generation of underwater or air vehicles, it is crucial to understand the role of fluid dynamics in animal locomotion. In this talk, I will focus on two highly maneuverable animals: knifefish and bats. A knifefish uses an electric field to “see” at night in the Amazon River, and it propels itself using a long ribbon fin to navigate around its complex environment. I will present experimental and computational work that unveils the mechanical basis of fin propulsion and the rich locomotor capability of these fish. In the second part of the talk, I will present a physical model to explore an open and controversial question in the evolution and origin of bat flight: how bats’ ancestors could have begun to employ flapping motions in their flight. I explore this question using a simple physical model which captures two key biological features: compliance and camber. The model is composed of a cantilevered flat plate (capturing the compliance) with a hinged trailing flap (modeling the variable camber). For slow wind speeds, the model is stationary, but above a critical wind speed, the wing starts to oscillate due to an aeroelastic instability. A positive angle of attack on the wing results in a positive lift force. Moreover, this lift force is significantly enhanced once the wing starts to oscillate. I will present particle image velocimetry (PIV) data to shed light on the aerodynamics of the self-excited flapping wing and to identify the mechanisms that generate the enhanced lift force. I will also discuss the implications of the results on the evolution of powered biological flight.
Thursday, March 8, 2012
11:00 AM
Laufer Library (RRB 208)
Helical Swimming in Complex Fluid Media
Bin Liu
Postdoctoral Research Associate
School of Engineering
Brown University
Providence, RI
Many bacteria swim by rotating helical flagella. In Nature, these cells often live in a complex fluid environment, such as suspensions of polymers and other micro-scale structures. To explore the physics on how such complex environments affect the bacterial motility, the helical swimmer is simulated by a model system – a motorized helical coil that rotates along its axial direction. When the helix is immersed in a viscoelastic fluid, a model fluid of polymer suspensions, there is an increase in the swimming speed as compared with the Newtonian case. The enhancement is maximized when the rotation rate of the helix matches the relaxation time of the fluid. The magnitude of enhancement depends not only on the elasticity of the fluid but also on the geometry of the helix. In the second part of my talk, I will discuss on how such helical swimming is affected by spatial confinement of micro scales, such as a porous medium. As a reduced order model, the porous media is regarded as cylindrical cavities with solid walls. A modified boundary element method is introduced here to make full use of the helical symmetry. This method allows us to investigate a situation that the flagella are tightly confined by solid wall. To our surprise, at fixed power consumption, a highly coiled swimmer swims faster in a narrower confinement, while an elongated one swims faster in a cavity
Wednesday, March 21, 2012
3:30 PM
Seaver Science Library, Room 150 (SSL 150)
Refreshments will be served at 3:15 pm.
How Do Flying Animals Reorient Themselves?
Attila Bergou
Postdoctoral Researcher
School of Engineering
Brown University
Providence, RI
The flying ability of animals is spellbinding: they can catch their prey in midair, execute precise maneuvers, sustain flight for incredible amounts of time. For centuries scientists and engineers have toiled to unlock the secrets behind their flight adeptness. This research has lead to many breakthroughs in our understanding of the behavior and force production of fixed and flapping wings and in no small part lead to the invention of the airplane. Despite the centuries of research, and many breakthroughs, fundamental aspects of animal flight still elude understanding. One domain with surprising gaps in knowledge is how animals actuate and control flight maneuvers.
Here, I will present experimental and computational work showing the mechanisms behind flight maneuvers performed by two decidedly distinct flyers: fruit flies, and bats. In particular, we analyze how these animals reorient themselves in the air. Our organismal experiments, and novel motion tracking algorithms allow us to reconstruct the subtle wing and body kinematics of these animals at a level of detail previously unavailable. From these intricate kinematics, and morphological measurements, we build detailed dynamical models to infer the surprising mechanisms behind these maneuvers.
Wednesday, March 28, 2012
3:30 PM
Seaver Science Library, Room 150 (SSL 150)
Refreshments will be served at 3:15 pm.
The Anatomy of Autonomy: An Aeronautical Systems Perspective
Michael S. Francis
Chief Advanced Programs & Senior Fellow
United Technologies Research Center
East Hartford, CT
and
Program Lead, Autonomous & Intelligent Systems
Sikorsky Aircraft Company
Stratford, CT
Enabled by the many byproducts of the information revolution, autonomous capabilities are rapidly redefining many of the industrial age platforms and processes created over the last century, while concomitantly enabling a host of new ones. This presentation examines the subject of autonomy and its role in shaping contemporary aeronautical systems, encompassing its genesis, implementation and evolution, as well as its potential in shaping the future. Key capabilities such as low altitude flight, collision avoidance, contingency management and multi-vehicle collaborative operations are featured to illustrate some of the discriminating elements enabled by autonomous functionality. Related topics such as the many ‘dimensions’ of autonomy, along with its conceptual underpinning; the importance of autonomous functionality in contemporary systems integration; and the nature and importance of the human-system interaction are also addressed. Examples involving advanced unmanned systems, including the Unmanned Combat Air System (UCAS) and the micro air vehicle are employed to further illustrate concepts and provide useful insights into the promise and limitations that frame today’s system designs. Key technical, institutional and cultural issues currently limiting realization of the potential of these systems are also discussed, as are some suggested ideas for their resolution.
Wednesday, April 4, 2012
3:30 PM
Seaver Science Library, Room 150 (SSL 150)
Refreshments will be served at 3:15 pm.
Bayesian Inference in Complex Physical Systems: Spectral Approximations and Optimal Maps
Youssef Marzouk
Professor
Department of Aeronautics and Astronautics
Massachusetts Institute of Technology
Cambridge, MA
Predictive simulation of complex physical systems increasingly rests on the interplay of experimental observations with computational models. Bayesian statistics provides a natural framework for quantifying uncertainty in parameter estimates and model predictions, for fusing heterogeneous sources of information, and for optimally selecting experiments or observations. Posterior simulation in Bayesian inference often proceeds via Markov chain Monte Carlo (MCMC), but the associated computational expense and convergence issues present significant bottlenecks in large-scale problems.
We present a new approach to Bayesian inference that entirely avoids Markov chain simulation, by constructing a map that pushes forward the prior measure to the posterior. Existence and uniqueness of a suitable measure-preserving map is established by formulating the problem in the context of optimal transport theory. We discuss various means of explicitly parameterizing the map and computing it efficiently through solution of an optimization problem, exploiting gradient information from the forward model when possible. The resulting scheme overcomes many of the computational bottlenecks associated with MCMC. Advantages of the map-based representation of the posterior distribution include analytical expressions for posterior moments and the ability to generate arbitrary numbers of independent posterior samples without additional likelihood evaluations or forward solves. The approach also provides clear convergence criteria for posterior approximation, and facilitates model selection through automatic evaluation of the marginal likelihood.
Wednesday, April 11, 2012
3:30 PM
Seaver Science Library, Room 150 (SSL 150)
Refreshments will be served at 3:15 pm.
Processing of Functional Nanocomposites for Laser, Thermoelectric and Renewable Energy Applications
Javier E. Garay
Associate Professor
and
Chair, Materials Science and Engineering Program
Mechanical Engineering Department
University of California, Riverside
Riverside, CA
Improved performance of devices often hinges on the development of materials with a precise blend of properties. In this talk, I will discuss our ongoing work on tailoring material properties using the platform of nanocrystallinity. Three different applications will be discussed: nanocrystalline materials for 1) improved thermoelectric performance, 2) increased laser power, and 3) improved permanent magnets for renewable energy generation. We will begin with an overview of the versatile material processing technique of current activated pressure assisted densification (CAPAD) which we use to overcome the grain growth challenge and efficiently produce materials large enough to be viable nanocrystalline parts. The method draws its effectiveness from large electric current densities that serve to heat the materials and also alter the processing kinetics. We will then discuss how precise control of nanostructure can lead to significantly improves properties for a wide range of applications. The materials produced are large-sized, fully dense materials with grain sizes much less than 100 nm. The results will be discussed in terms of crystal length scale effects and proximity of nanoscale phases.
Thursday, April 12, 2012
11:00 AM
Laufer Library, (RRB 208)
Refreshments will be served at 10:45 am.
Computational and Experimental Study of Laminar Flames
Mitchell D. Smooke
Strathcona Professor of Mechanical Engineering & Materials Science & Applied Physics
Department of Mechanical Engineering
Yale University
New Haven, CT 06520-8284
As emissions legislation becomes more restrictive, a detailed understanding of pollutant formation in flames has become even more critical for the design of pollutant abatement strategies and for the preservation of the competitiveness of combustion related industries. It is clear that there will be continuing pressure to lower both NOx emission indices and soot volume fractions in practical combustion devices. This is in response to the toxicological effects of small particles and to the impact, for example, that soot can have on thermal radiation loads in combustors and on turbine blades. Moreover, soot emissions can enhance contrail formation and such “man made” clouds may have an impact ultimately on the Earth’s climate. In this talk we discuss our research related to the effects of complex chemistry and detailed transport on the structure and extinction of hydrocarbon flames in coflowing axisymmetric configurations. We have pursued both computational and experimental aspects of the research in parallel on both steady-state and time-dependent systems. The computational work has focused on the application of accurate and efficient numerical methods for the solution of the steady-state and time-dependent boundary value problems describing the various reacting systems. Detailed experimental measurements were performed using two-dimensional imaging techniques. Our goal has been to obtain a more fundamental understanding of the important fluid dynamic and chemical interactions in these flames so that this information can be used effectively in combustion modeling.
Wednesday, April 25, 2012
3:30 PM
Seaver Science Library, Room 150 (SSL 150)
Refreshments will be served at 3:15 pm.
Fall, 2012
Aero-Hydrodynamics and Dynamics of Air-Assisted Marine Vehicles
Konstantin Matveev
Associate Professor
School of Mechanical and Materials Engineering
Washington State University
Pullman, Washington
Konstantin Matveev obtained a Master degree in Applied Physics from Moscow Institute of Physics and Technology in 1996 and a Ph.D. degree in Mechanical Engineering from California Institute of Technology in 2003. His Ph.D. thesis was on thermoacoustic and combustion instabilities. As a postdoc at Los Alamos National Laboratory, Dr. Matveev investigated nonlinear thermoacoustic phenomena in advanced energy systems. As a senior hydrodynamicist at Art Anderson Associates and a consultant to several European R&D organizations, he was involved in the development of high-performance marine concepts, including air-lubricated hulls, hydrofoils, multi-hull ships, and wing-in-ground craft. He recently co-authored a technical book on Small-Waterplane-Area Ships. At the present time, Dr. Matveev is an Associate Professor in the School of Mechanical and Materials Engineering at Washington State University. His current research is focused on advanced marine vehicles and thermoacoustics.
Wednesday, September 5, 2012
3:30 PM
Seaver Science Library, Room 150 (SSL 150)
Refreshments will be served at 3:15 pm.
Application of Laser and Optical Diagnostics for Study of Next Generation Propulsion Systems and Integration of Alternative Bio-derived Fuels
Tonghun Lee
Associate Professor
Department of Mechanical Engineering
Michigan State University
East Lansing, Michigan
Development of advanced propulsion systems of the future, such as hypersonic ramjets and pulsed detonation engines will require a deeper understanding of the reactive flow dynamics and chemistry in the relevant turbulent regime as well as development of new energetically enhanced combustion technologies. Additionally, these new engines will require seamless operation across a wide range of new and alternative bio-derived fuels as well as conventional hydrocarbon counterparts. This presentation will discuss recent progress in applying laser and optical diagnostics to study high Reynolds number reactive flows, plasma enhanced flames, as well as ignition and oxidation of alternative bio-derived jet fuels for potential integration in next generation propulsion systems. The following three topics will be discussed.
First is an effort to develop a continuous high-speed (>10 kHz) laser imaging system which integrates PLIF, PIV, and Rayleigh Scattering Thermometry (RST) for detection of temperature fields as well as velocity and chemical species. The proposed work will increased the number of detectable species in reactive flows by extending the wavelength range further into the UV with enhanced beam stability through non-linear optical engineering. Second is a novel concept of energetically enhancing the combustion by using a non-equilibrium plasma discharge. Highly efficient flame stabilization is achieved using a non-equilibrium plasma discharge by coupling the plasma energy directly into the reaction zone of the flame. The electrons and ions generated by the plasma introduce new chemical pathways which enhance and accelerate the combustion chemistry. The third topic of discussion is investigating the ignition and oxidation characteristics of bio-derived jet fuels. A rapid compression machine is modified for ignition testing of heavy fuel blends using a ‘direct test chamber’ fuel preparation method as well as optical access for laser and optical diagnostics.
Tonghun Lee is an associate professor in the Department of Mechanical Engineering at Michigan State University. Prior to working at Michigan State University, he was a graduate student at Stanford University where he obtained his M.S. (2002) and Ph.D. (2006) degrees in the ‘High Temperature Gas Dynamics Laboratory.’ His research is focused on utilizing laser and optical diagnostics to investigate new combustion concepts in advanced propulsion and energy conversion systems, with particular emphasis on supersonic air-breathing engines and high-pressure combustion research. He has been recognized with numerous awards including the AFOSR Young Investigator Award, the ONR Young Investigator Award, and the Presidential Early Career Award for Scientists and Engineers (PECASE).
Wednesday, September 12, 2012
3:30 PM
Seaver Science Library, Room 150 (SSL 150)
Refreshments will be served at 3:15 pm.
Hierarchical Architected Materials as a Platform for Novel Multifunctional Systems
Lorenzo Valdevit
Assistant Professor
Mechanical and Aerospace Engineering Department
University of California, Irvine
Irvine, CA 92697
Periodic cellular materials are desirable for applications requiring high specific stiffness and strength. If the architecture is open-celled, a number of multifunctional attributes can be added, with potential for multi-objective optimization. Although conventional industrial technologies limit the achievable unit cell complexity, recent progress in advanced manufacturing is enabling fabrication of hierarchical cellular materials of nearly any topology with wide dimensional bandwidth (i.e., the ratio of the dimension of the largest to the smallest feature in the architecture).
Hierarchical unit cell designs with wide dimensional bandwidth are showing particular promise, often revealing unique mechanical behavior. Furthermore, if the smallest feature in the architecture is at the sub-micron scale, unique size effects in plasticity can further improve the mechanical response.
In this presentation, I will discuss novel ultra-light micro-architected nickel hollow-truss lattices with unprecedented combinations of density, stiffness, strength and damping characteristics. Results of extensive experimental investigations (both at the nano and macro-scale) will be presented, alongside numerical models and optimal design tools. Some preliminary work on ceramic and hybrid architected materials manufactured by 3D printing will also be discussed. Collectively, all these investigations indicate that a strong synergism among advanced manufacturing, materials science, multi-scale experimental and computational mechanics and sophisticated optimization tools is required to reach the full potential of hierarchical architected materials.
Lorenzo Valdevit received his MS degree (Laurea) in Materials Engineering from the University of Trieste, Italy (in 2000) and his PhD degree in Mechanical and Aerospace Engineering from Princeton University (in 2005). He worked as an intern at the IBM T.J. Watson Research Center and as a post-doctoral scholar at the University of California, Santa Barbara. He is presently an Assistant Professor in the Mechanical and Aerospace Engineering Department at the University of California, Irvine (with a joint appointment in Chemical Engineering and Materials Science). He is a member of Pi Tau Sigma and Tau Beta Pi and is the recipient of the 2007 Faculty Award from IBM Corporation. His primary research goal is the optimal design, fabrication and experimental characterization of micro-architected materials with superior combination of properties. His group has recently developed novel micro-mechanical test frames and numerical algorithms to help achieve this overarching goal.
Wednesday, September 19, 2012
3:30 PM
Seaver Science Library, Room 150 (SSL 150)
Refreshments will be served at 3:15 pm.
From the Atoms Up: Design and Deformation of Metallic Glasses and their Composites
Katharine M. Flores
Professor and Associate Chair
Department of Mechanical Engineering and Materials Science
and
Associate Director
Institute of Materials Science and Engineering
Washington University
St. Louis, MO
Metallic glasses, metallic alloys with disordered atomic structures, have matured from laboratory curiosities to engineering alloys of interest for their remarkable mechanical properties and processability. The unique atomic structure of these alloys presents a number of challenges for describing and controlling their behavior, as well as intriguing manufacturing opportunities. Lacking long-range structural order and the attendant microstructure, metallic glasses do not require multiscale modeling to capture many essential characteristics of their behavior and thus are an ideal model system for the development of a computationally driven “atoms-up” approach to alloy design. We use molecular dynamics to determine the liquid fragility for a series of Cu-Zr alloys and discuss this measure of the dynamics of the system in light of observations of the short-range atomic ordering of the alloy. Based solely on these parameters calculated from molecular dynamics, we suggest an a priori predictor for glass forming ability.
While monolithic metallic glasses exhibit near theoretical strengths and large elastic deflections, their lack of extensive tensile ductility limits their structural applications. Microstructural control has long been the materials scientist’s chief tool for improving material properties. By creating metallic glass-crystalline composites, we introduce microstructural features and gain control over the initiation and distribution of plastic deformation. Building upon our computationally-driven approach to the design of the glassy phase, we next seek to design metallic glass composites with desirable properties. This requires adequate understanding of the role of microstructure on the plastic deformation mechanisms in order to calibrate and validate the model. One family of composites utilizes ductile crystalline dendrites which precipitate from the melt prior to vitrification of the matrix. Our work to quantitatively characterize this microstructure and its role in the resulting mechanical behavior will be discussed. Full field strain mapping measured from in situ digital image correlation reveals that at relatively low strains, the glassy matrix acts as an isotropic “buffer layer” between crystals of different orientations, permitting the crystals to deform unimpeded along their preferred slip system.
Katharine Flores is Professor and Associate Chair of Mechanical Engineering and Materials Science at Washington University, as well as the Associate Director for the new interdisciplinary Institute of Materials Science and Engineering. After receiving her B.S. in Mechanical Engineering from Washington University in 1995, she attended Stanford University where she received her Ph.D. in Materials Science and Engineering in 2000. She joined the Materials Science and Engineering Department at the Ohio State University as an assistant professor in 2002, and moved to her current position at Washington University in July, 2012. Her primary research interest is the mechanical behavior of structural materials, with particular emphasis on understanding structure-processing-property relationships in bulk metallic glasses and their composites, an area in which she has worked for almost 15 years. She leads research projects on topics ranging from investigations of the structural origins of plastic deformation in metallic glasses to the design of new glasses with desirable properties and the development of new manufacturing techniques suited to the unique processing capabilities of these alloys, in an effort to accelerate their incorporation in mainstream and high performance applications. In 2005, she received two prestigious early-career awards for her work in this area, a National Science Foundation CAREER Award and an Office of Naval Research Young Investigator Award. In addition to her research, Dr. Flores is actively involved in outreach and was the Director of Education and Outreach for the Center for Emergent Materials, the NSF Materials Research Science and Engineering Center at OSU, from 2008-2012. In 2011, she was a co-recipient of an Ohio Faculty Innovator Award for her efforts to improve undergraduate instruction in materials science and engineering.
Wednesday, September 26, 2012
3:30 PM
Seaver Science Library, Room 150 (SSL 150)
Refreshments will be served at 3:15 pm.
Serendipity and Contrarianism in Research: Hit ‘Em Where They Ain’t
Paul Ronney
Professor
Department of Aerospace & Mechanical Engineering
University of Southern California
Los Angeles, CA
Almost every great scientific discovery involves serendipity, i.e. a chance encounter with unexpected results. This is no accident, for if one already expects a particular result and research confirms the expectation, nothing fundamentally new was learned. Examples include Teflon™, radioactivity, nitrocellulosic explosives, LSD, the Big Bang and the microwave oven. In each of these cases, when unexpected results presented themselves, the investigators were able to identify their significance instead of merely dismissing them as failures. Frequently another key aspect of discovery is contrarianism, that is, the ability and commitment to challenge accepted wisdom in order to resolve its weaknesses or contradictions. Examples include quantum mechanics, relativity and anthropomorphically-induced climate change. Contrarianism may also take the form of a simpler solution to solving a demanding problem, e.g. tetraethyl lead anti-knock additive for gasolines.
This seminar presents my experiences (which pale in significance compared to the aforementioned cases) with serendipity and contrarianism in the context of research on chemically reacting flows, including examples from microgravity combustion, turbulent flames, catalysis, photobleaching velocimetry and biophysics. Most importantly, I will provide suggestions to fledgling researchers (i.e., graduate students) on how to identify serendipitous yet consequential results and when/how to stand firm in the face of adversity resulting from contrarianistic views.
Wednesday, October 3, 2012
3:30 PM
Seaver Science Library, Room 150 (SSL 150)
Refreshments will be served at 3:15 pm.
Applications of “Bottom Up” Multiscale Modeling for Polymer Self Assembly and Protein-Surface Binding
Jim Pfaendtner
Assistant Professor
Chemical Engineering Dept.
University of Washington
Seattle, WA
Computational models such as molecular dynamics (MD) hold great potential for connecting the atomic scale to the mesoscale for a wide range of problems of engineering interest. Unfortunately, severe computational restrictions often limit wide-ranging use of these tools. New multiscale modeling algorithms that are based on MD have been developed that can overcome these challenges, dramatically increasing the computer’s viability as a tool for computation-driven discovery. The first part of this talk will highlight how we are using the computer to study the self-assembly of conjugated polymers in solution. The model polymer poly(3-hexylthiophene) has been studied in a variety of solvents. Solvent and temperature-dependent trends are extracted to study the impact of solvation and polymer architecture on intra-chain and inter-chain interactions. The second part of the seminar will investigate how simulations can be used to study thermodynamic driving forces that lead to self-assembly of peptide monolayers on surfaces.
Jim Pfaendtner holds a B.S. in ChE (GA Tech, 2001) and a PhD in Chemical Engineering (Northwestern University, 2007). He joined the faculty of University of Washington in 2009 as an assistant professor. Prior to joining the UW he received an NSF IRFP postdoctoral fellowship to work under the supervision of Prof’s Greg Voth and Michele Parrinello. Jim is the recipient of a 2012 NSF CAREER award and is a 2012 Kavli Fellow of the US National Academy of Science. Jim’s research group focuses on development and application of computational tools for multiscale modeling and simulation of soft matter systems.
Wednesday, October 10, 2012
3:30 PM
Seaver Science Library, Room 150 (SSL 150)
Refreshments will be served at 3:15 pm.
Magnetic Resonance Imaging for Measurement of Velocity and Concentration in Complex Turbulent Flows
John K. Eaton
Charles Lee Powell Foundation Professor of Engineering
Mechanical Engineering Department
Stanford University
Stanford, CA
Magnetic Resonance Imaging (MRI) based flow measurement techniques are enabling a paradigm shift in how we study, evaluate, and design turbulent flows in complex geometries. MR Velocimetry (MRV) measures a complete 3-D mean velocity field and does not require flow tracers or optical access. Resolutions as fine as 0.25 mm have been achieved in measurement volumes as large as 100 X 100 X 250 mm. An entire velocity field comprising millions of individual data points can be measured in a few hours. When combined with computer-aided design and rapid-prototyping of the flow model, a complete mapping of a flowfield from conception to data analysis can be completed in a week. This versatile technique has been used to map the external flow around a coral colony, internal flows in serpentine passages, separated flows in three dimensional diffusers, and the combination of flows found in a full combustor geometry. The detailed 3D measurements allow understanding of vortex structures which dominate many flows, the interplay between separation zones, and analysis of flow splits among parallel paths. A second technique called Magnetic Resonance Concentration (MRC) provides quantitative measurements of the time-averaged 3D concentration field for passive scalar mixing. The combination of measured 3D concentration and velocity fields provides enough detail to yield exceptional understanding of the effects of mean flow transport and turbulent mixing. This has proved particularly effective in the iterative design of film-cooling and other systems where we wish to suppress turbulent mixing. In addition, the combined velocity and concentration data are being used to characterize and improve numerical models for turbulent mixing.
John K. Eaton is the Charles Lee Powell Foundation Professor of Engineering at Stanford University where he has been on the faculty since 1980. He earned all his degrees at in Mechanical Engineering at Stanford. He conducts research in turbulence, convective heat transfer, advanced measurement techniques, multiphase flow, and flow through random media. Recent emphasis has been on high-fidelity, rapid turnaround experiments in complex flows, measurement and modeling of turbulent mixing, and extreme sensitivity of certain high Reynolds number flows to small perturbations. Much of Professor Eaton’s work is motivated and funded by problems in the gas turbine industry. He has supervised 49 completed Ph.D. dissertations including those of 15 professors. He has won both the Tau Beta Pi and Perin Awards for teaching excellence, was an NSF Presidential Young Investigator Awardee, and is a Fellow of the American Society of Mechanical Engineers and the American Physical Society.
Wednesday, October 17, 2012
3:30 PM
Seaver Science Library, Room 150 (SSL 150)
Refreshments will be served at 3:15 pm.
Dynamic Response of Sandwich Composites Subjected to Shock Loading
Arun Shukla
Simon Ostrach Professor
Department of Mechanical, Industrial and Systems Engineering
University of Rhode Island
Kingston, RI 02881
This lecture will present studies on the response of novel engineering layered materials to extreme dynamic loadings. In particular, the talk will focus on the behavior of sandwich composite materials to shock loading. A shock tube facility was utilized to apply blast loadings to simply supported plates of sandwich composite materials. Pressure sensors were mounted at the end of the muzzle section of the shock tube to measure the incident and the reflected pressure profiles during the experiment. This data was used to evaluate the energy transferred and the impulse imparted to the sandwich structure. Three high speed digital cameras along with 3D digital image correlation technique were utilized to capture real time deformation of sandwich composites, as well as the development and progression of damage in them. Based on physical observations better sandwich panels were designed for blast mitigation.
Arun Shukla obtained a Bachelor of Technology degree from the Indian Institute of Technology (IIT), Kanpur and his Master of Science and Doctorate degrees from the University of Maryland, all in Mechanical Engineering. He joined the University of Rhode Island in 1981 as a faculty member and currently serves as the Simon Ostrach Professor in the Department of Mechanical, Industrial and Systems Engineering. He has also served as the Clark. B Millikan Visiting Professor at the California Institute of Technology, Visiting Professors at the Indian Institute of Technology and Texas A&M Universities and as Design Engineer at Voltas Limited.
He is a Fellow of the Society for Experimental Mechanics, American Academy of Mechanics and American Society for Mechanical Engineers. He was elected to the European Academy of Sciences and Arts in 2011. He has received the Frocht, Lazan, Taylor and Tatnall Awards and Murray Medal from the Society for Experimental Mechanics. At the University of Rhode Island, he is a recipient of the Simon Ostrach First Endowed Professorship Award, the Vincent and Estelle Murphy Faculty Excellence Award, Distinguished Engineering Professor Award, the University’s Scholarly Excellence Award, the Albert E Carlotti Faculty Excellence Award and the University’s Outstanding Research Achievement Award. He received the Distinguished Alumnus Award from IIT, Kanpur in 2009.
In 2003, he served as the President of the Society for Experimental Mechanics. He has served as the Technical Editor of the journal Experimental Mechanics. He has also served the National Research Council, on the United States National Committee on Theoretical and Applied Mechanics. Along with his many graduate students and post docs, Professor Shukla has published more than 300 papers in refereed journals and proceedings. He has also co-authored and edited 5 books, and has delivered numerous plenary and keynote lectures.
Wednesday, October 24, 2012
3:30 PM
Seaver Science Library, Room 150 (SSL 150)
Refreshments will be served at 3:15 pm.
Bird Design and Aircraft Evolution
Geoff Spedding
Professor and Chairman
Aerospace & Mechanical Engineering Department
University of Southern California
Los Angeles, CA
Wednesday, October 31, 2012
3:30 PM
Seaver Science Library, Room 150 (SSL 150)
Refreshments will be served at 3:15 pm.
Micro- and Nano-systems for Extreme Harsh Environments
Debbie G. Senesky
Assistant Professor
Aeronautics and Astronautics Department
Stanford University
Stanford, CA
Wide bandgap semiconductor materials are inherently temperature-tolerant, radiation-hardened and biocompatible which can extend the operation regime of micro- and nano-scale devices to extreme harsh environments (e.g. deep space, subsurface environments, combustion environments, and the human body). In addition, wide bandgap semiconductor materials are often simultaneously piezoelectric, piezoresistive and pyroelectric, which can be leveraged in the design of a multitude of micro- and nano-scale devices such as inertial sensors, bolometers, micromechanical resonators and energy harvesters in a single material layer. In this talk, a review of the advancements in manufacturing technology for polycrystalline thin film, epitaxial thin film and nanowire growth of wide bandgap semiconductor materials is presented. In addition, the compelling results of silicon carbide (SiC), gallium nitride (GaN), and aluminum nitride (AlN) device operation at temperatures as high as 600°C will be reviewed. These robust material sets can serve as a platform for the realization of sensor, actuator and electronic systems that can operate and collect data under the most hostile conditions.
Debbie G. Senesky received the B.S. degree in mechanical engineering from the University of Southern California in 2001. She received the M.S. and Ph.D. degrees in mechanical engineering from the University of California, Berkeley in 2004 and 2007, respectively. From 2007 to 2008, she was a Design Engineer for GE Sensing (formerly known as NovaSensor). From 2008 to 2012, she was a postdoctoral researcher at the Berkeley Sensor and Actuator Center developing silicon carbide (SiC) sensing technology for extreme harsh environments. Recently, she has been appointed to the faculty in the Aeronautics and Astronautics Department at Stanford University. Her research interests include the development of micro- and nano-scale sensors, wide bandgap electronics and ceramic materials for operation within extreme harsh environments.
Wednesday, November 7, 2012
3:30 PM
Seaver Science Library, Room 150 (SSL 150)
Refreshments will be served at 3:15 pm.
Amplifiers and Oscillators: A Hydrodynamic Stability Perspective
Patrick Huerre
Hydrodynamics Laboratory — LadHyX
CNRS — Ecole Polytechnique
France
Complex flows of interest in aeronautical applications may often be considered as involving a combination of simpler prototypical free shear flows such as mixing layers, wakes, jets, etc. which prevail in different spatial subdomains. Such archetypical shear flows are in a turbulent state but they are known to exhibit large-scale vortical structures which dominate the dynamics up to very large Reynolds numbers. Experimental observations indicate that some flows are extrinsic amplifiers of external noise while others are intrinsic oscillators which beat at a specific frequency. In this lecture, such structures will be described as instablity waves living on a suitable mean flow. Hydrodynamic stability concepts such as convective/absolute instability, local/ global instability, will then be shown to account for the extrinsic or intrinsic spatio-temporal dynamics of coherent structures in several shear flows. The classical locally parallel asymptotic analyses and the more recently developed fully non-parallel numerical approaches will be critically assessed.
Wednesday, November 14, 2012
2:00 PM
Doheny Library, Room 240 (DML 240)
Refreshments will be served following
Rotation Effects on Instability and Turbulence on Simple Shear Flows
P. Henrik Alfredsson
Linné FLOW Centre
KTH Mechanics
Royal Institute of Technlogy
Stockholm
Sweden
Effects of rotation (centrifugal and/or Coriolis forces) are usually felt on large scales (such as planetary scales) or at very high rotation rates such as turbines and compressors. However laboratory scale shear flows may be strongly affected also by quite small rotation rates. In this talk we concentrate on two cases: spanwise rotation of shear flows (here we will focus on plane Couette flow) and axial rotation of circular pipe flow. Both flows show surprising effects of the rotation, in the first case due to the Coriolis force that can either stabilize or destabilize the flow. For stabilizing rotation turbulence may be completely killed, whereas for the destabilizing case a number of different instabilities can be observed. For the axially rotating turbulent pipe flow the symmetry is broken by rotation and the flow will never reach solid body rotation. An unexpected feature occurring if the flow is issued as a swirling jet will also be discussed.
Friday, November 16, 2012
2:00 PM
Grace Ford Salvatori Hall, Rm. 101 (GFS 101)
Progress, Challenges, and Opportunities in 3-D Patient-Calibrated Computational Modeling of Cancer
Paul Macklin
Co-Director
Consortium for Integrative Computational Oncology (CICO)
and
Assistant Professor of Research Medicine
Center for Applied Molecular Medicine
Keck School of Medicine
University of Southern California
Los Angeles, CA
Clinical oncology practice can generate a wide variety of data for patients, spanning molecular profiling, pathology, and radiology, which tend to be used in isolation when planning patient care. There is currently no technique to quantitatively combine these diverse data sources, along with novel in vitromeasurements, to improve surgical and therapeutic planning. Similarly, while cancer research generates a staggering wealth of data spanning many scales, these data are generally not integrated into comprehensive models. We are likely missing emergent system-level cancer phenomena and the novel therapeutic targets they represent. In this talk, we will discuss efforts by my lab, the USC Physical Sciences Oncology Center, and the Consortium for Integrative Computational Oncology to solve these issues. With a focus on patient pathology-calibrated breast cancer modeling and multidisciplinary modeling of liver metastases, we will discuss the role for computational modeling in cancer research, encouraging results in quantitatively predicting clinical progression, the implications for making and quantitatively testing biological hypotheses, and the role of computational modeling in facilitating a deeper understanding of biology, pathology, and radiology. More information can be found at MathCancer.org.
Wednesday,November 28, 2012
3:30 PM
Seaver Science Library, Room 150 (SSL 150)
Refreshments will be served at 3:15 pm.
Design and Testing of Advanced Wind Turbine Technologies
Pier Marzocca
Associate Professor
Department of Mechanical & Aeronautical Engineering
Clarkson University
Potsdam, NY
New advanced composite blades design methodologies can significantly impact the performance and reliability of wind turbine technologies. Current approaches employed in designing composite turbine blades, resort to sophisticated multi-physics codes taking into account fluid-structure interaction. When it comes to structural health monitoring and damage progression, the practitioner needs to evaluate the integrity of the composite structure by using in situ real-time techniques, often neglecting the effect of the degradation of structure properties, particularly when addressing complex aeroelastic simulations. The prediction of damage progression in composite wind turbine blades under dynamic aeroelastic conditions is usually a cumbersome multi-step process with significant manual user intervention. The talk with discuss a novel approach where the different components of this process, the dynamical structural analysis under varying aerodynamic and deterministic loads, and damage progression, are integrated into one reduced-order model capable of predicting the occurrence and progression of damage in real time. Severe load cases and the potential for real-time predictions of damage progression will be discussed. Several aspects of current wind turbine projects including modeling and experimental investigations will be discussed.
Pier Marzocca has been a faculty member in the Mechanical and Aeronautical Engineering Department at Clarkson since 2003. He received his doctoral degree in Aerospace Engineering from Politecnico di Torino, Italy, and worked as a Postdoctoral Researcher and Visiting Assistant Professor in Engineering Science and Mechanics at Virginia Tech before joining Clarkson University. Professor Marzocca has been working in the field of aerospace engineering since 1996 and specializes in multi-physics modeling and characterization of advanced materials and structures, dealing with the interactions among advanced structures and fluids, magnetic, electric, and thermal fields. His research interests reside in structural dynamics and controls of advanced aerospace systems, including active flow and aeroelastic controls of flexible structures. Dr. Marzocca has received several awards including the Ralph R. Teetor Educational Award from the SAE. He is currently an Associate Fellow of AIAA, the Chair of the SAE Unmanned Aircraft System Technical Committee, Deputy Editor-in-Chief of the International Journal of Aeronautical and Space Sciences, and Associate Editor of the ASCE Journal of Aerospace Engineering and the Journal of Thermal Stresses.
Wednesday, December 5, 2012
3:30 PM
Seaver Science Library, Room 150 (SSL 150)
Refreshments will be served at 3:15 pm.
Published on September 8th, 2017
Last updated on October 4th, 2017